Severe winter weather events cause numerous infrastructure and building failures throughout the United States that can lead to medical emergencies for occupants and millions of dollars in damage. Winter weather preparedness and adoption of winter weather resiliency measures can greatly reduce the risk of emergencies and property damage.
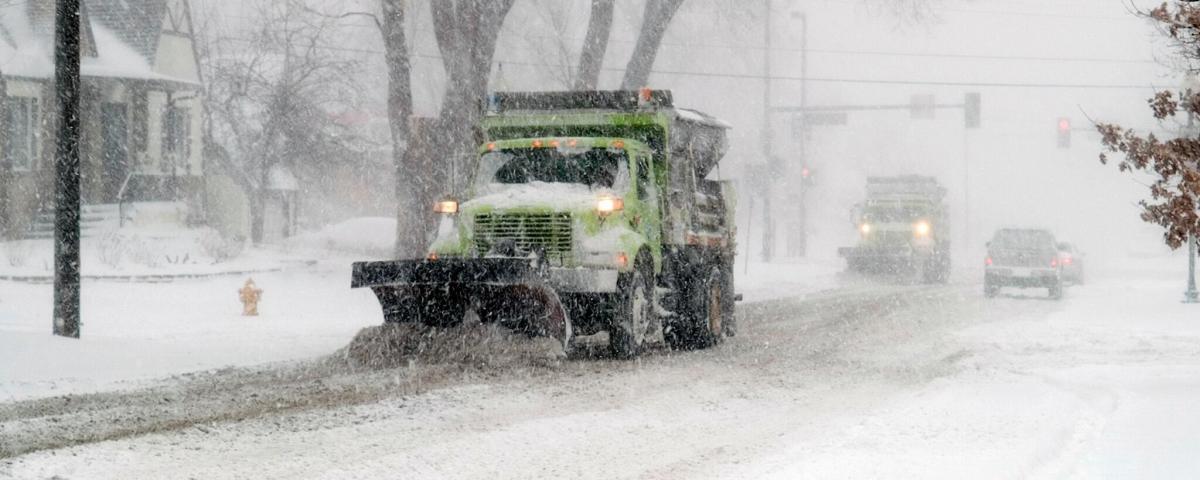
This guide provides a general overview of strategies to improve occupant safety and minimize property damage; topics covered include
- understanding severe winter weather event hazards
- understanding snow loads
- designing and retrofitting for thermal resiliency
- planning for winter weather events.
This overview guide is intended to help homeowners, contractors, and builders assess severe winter weather event risks and consider mitigation measures for one- and two-family dwellings. Detailed guidance on specific topics related to winter weather resistance can be found in the guides attached to the winter weather resistance guide list.
Understanding Severe Winter Weather Event Hazards
Building code amendments are state specific; what is considered severe winter weather in one region of country may not be considered severe in another region. In this guide, severe winter weather is characterized as any winter event with the potential to cause building system failures or a threat to human health in a minimally code-compliant home for that climate zone. Climate-zone specific guidance in building codes for insulation levels, snow loads, and plumbing protection is based on historical averages. As weather patterns change, buildings may be put under more stress than they were originally designed for. Power outages during a winter storm are especially damaging, as thermal resiliency strategies are not often mandated by local codes. The most common hazards associated with severe winter weather events are:
- carbon monoxide poisoning
- house fires
- loss of electricity and heat
- frozen and damaged plumbing
- roof collapse.
Carbon monoxide poisoning cases and incidences of house fires both rise significantly during severe winter weather events (Figure 2), generally as a result of occupants attempting to heat their homes using unsafe means during power outages. Fireplaces that have not been maintained for long periods of time or that are left unattended through the night and the operation of gas cooking appliances, grills, and generators within the home or automobiles in closed garages to try to stay warm all contribute to deaths and an increase in emergency room visits during winter storm events. While these are primarily issues of occupant education, there are steps contractors can take to design or retrofit with the mitigation of these preventable hazards in mind. Methods for designing and retrofitting buildings to add resilience during winter power outages and heavy snow events are outlined below.
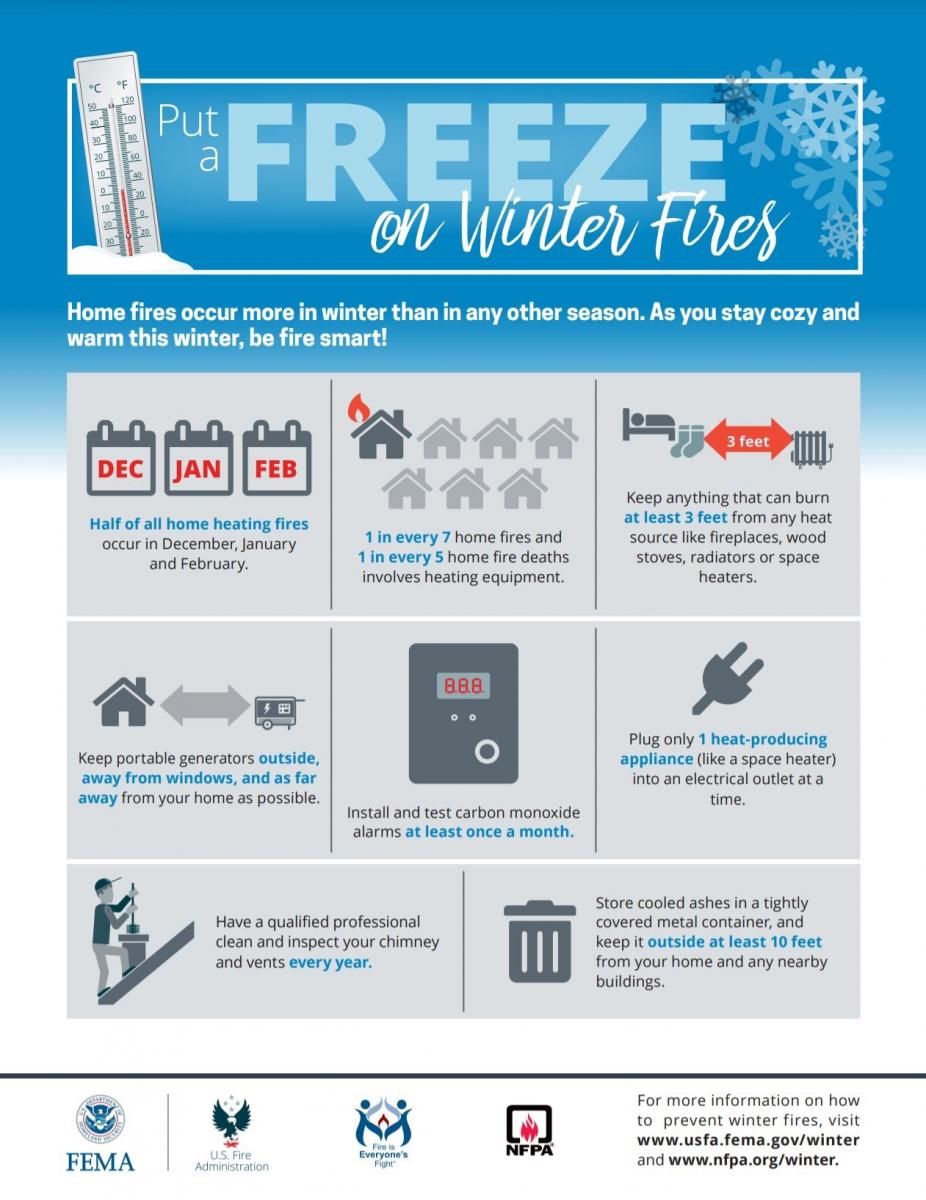
Understanding Snow Loads
Criteria related to snow loads, frost line depth, and ice barrier underlayment are specified in Chapter 3 of the International Residential Code. The IRC (2021), Section R301.2.3 “Snow Loads” notes that homes built in regions with ground snow loads of 70 pounds per square foot or less should be constructed in accordance with Chapters 5, 6, and 8 of the IRC while in regions with ground snow loads greater than 70 pounds per square foot, homes should be designed in accordance with accepted engineering practice. Ground snow loads for the United States are indicated in IRC 2021, Figure R301.2(3 and 4).
Snow loads vary widely across North America, from 10 to 20 pounds per square foot in the southern climate zones, to 100 pounds per square foot in parts of New England and the Western Great Lakes regions, and as high as 300 pounds per square foot in the northern rainforest regions of Alaska and Western Canada. In addition to the quantity of snowfall, the other significant factor contributing to this variation between snow loads is the moisture content of snow in different regions. Maritime regions will generally have snow with higher moisture content than arid regions with continental climates. The moisture content of snow ranges between ~1% to ~33%. The age of snow also affects its moisture content, as compressed snow that accumulates over time will weigh more than freshly fallen snow. Windborne snow drifting can also affect weight. In locations with fluctuating temperatures, where snow may melt off roofs periodically throughout the winter during warm periods, the largest threat to roofs tends to occur during large snow events. In colder regions, where snow accumulates over the course of the winter but does not periodically melt, the end of the winter is when the cumulative snowfall and condensed older snow is likely to cause the most structural failures in roofs.
Snow loads on roofs and decks can be based on the ground snow load for the region or by using a measurement called Snow Water Equivalent. Ground snow loads are based on regional 50-year mean recurrence intervals, or 2% probability of being exceeded in any given year. Building codes in different regions of the country will consider the ground snow load and may add a factor of safety to it. The American Society of Civil Engineers (ASCE) publishes a map with the Ground Snow Loads for different regions of the country. ASCE guidance on determining snow loads (as well as dead, live, soil, earthquake, wind, flood, tsunami, rain, and atmospheric ice loads) is specified in ASCE/SEI 7-16: Minimum Design Loads and Associated Criteria for Buildings and Other Structures. This standard is described in the ASCE document Snow Loads: Guide to the Snow Load Provisions of ASCE 7-16 (Michael O’Rourke 2017).
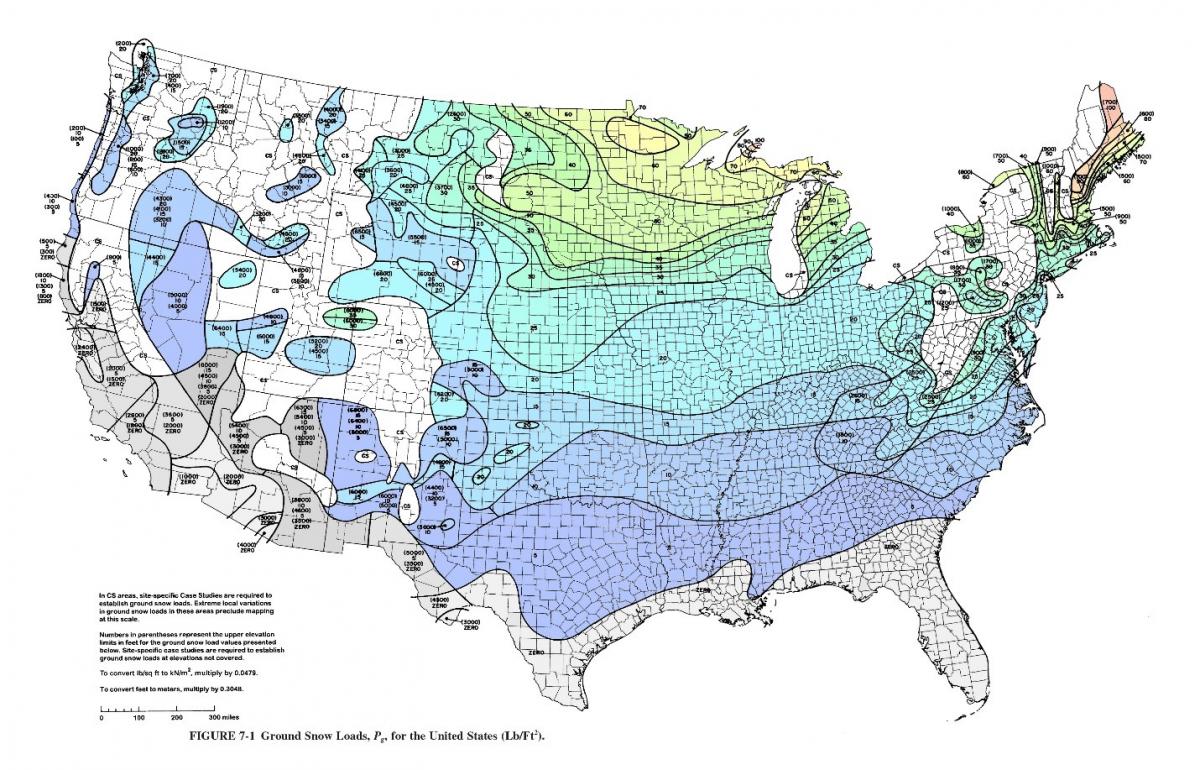
In certain parts of the country, such as the Mountain West, the Appalachian and Adirondack Mountains, and the southern Great Lakes, local variations are extreme enough that the ASCE map is insufficiently precise, and site-specific case studies are used instead, as noted in the footnote on the map above from the ASCE 7-10.
When calculating snow load by using the snow water equivalent, a cylindrical core sample of snow from a specific area is melted and converted from inches of snow to inches of water to obtain the snow water equivalent. The U.S. Department of Agriculture’s Natural Resources Conservation Service (NRCS) National Water and Climate Center tracks snowpack sensor stations at multiple sites across the country. The center’s Snow Telemetry (SNOTEL) and snow course information can be found here: Snow Telemetry (SNOTEL) and Snow Course Data. To calculate pounds per square foot, the snow water equivalent is multiplied by a conversion factor of 5.2.
Building codes use historical data to mandate minimum design loads for roofs. However, as weather patterns change and codes are updated, existing structures are not required to be retrofitted to meet the new codes. That means that older structures may be more prone to collapse than more recently constructed buildings. Buildings built offsite and then transported to different regions may also be at risk. Manufactured homes, for instance, can be built to carry just 20 psf but could be located in areas with heavier snow loads.
Roof snow loads can vary due to roof type, slope, and venting. Flat roofs are generally not appropriate for residential structures in regions with high snow loads. Pitched roofs, while less prone to collapse than flat roofs, may shed snow suddenly. If snow-shedding paths from pitched roofs are not considered during the design phase, shedding snow has the potential to cause damage or even human harm. Falling and sliding snow can damage decks, chimneys, roof vents, parked cars, HVAC equipment, and can injure or even kill people. Contractors and designers should consider sliding snow deposit zones and ensure that snow shedding from the roof has a safe path to the ground without the potential to cause harm to occupants or damage property. Contractors should also consider how additions or modifications to existing buildings may be affected by both snow load and shedding snow. For instance, an addition that is code compliant but sheds additional snow onto older parts of the structure that may not be up to the current code may cause unexpected damage. Solar panels and solar thermal arrays should also be arranged so that melting snow does not slide off in a direction that will damage property.
Designing and Constructing Buildings for Thermal Resiliency
One of the primary things builders can do to improve the winter weather resiliency of a home is to construct the home with good air sealing, good insulation, and good water management. A thermal envelope designed to lower one’s energy bills will also make a home more resilient to extreme winter weather events by maintaining interior temperatures longer without having to operate mechanical systems. In new construction, designers and builders must plan to meet or exceed the energy code in their state. For increased winter weather resistance, follow the envelope requirements outlined in the federal above-code home labeling programs DOE Zero Energy Ready Home (Figure 4) and ENERGY STAR Certified Home. Installers guides to meet the requirements of these two programs are linked to checklists that can be found in the Building America Solution Center at the following:
- U.S. Department of Energy’s Zero Energy Ready Home program
- ENERGY STAR Certified Home program
In existing homes, air sealing penetrations, adding insulation to attics, basements, and crawlspaces; and upgrading windows by installing ENERGY STAR rated low-e storm windows or replacing existing single- or double-pane windows with triple-pane windows can greatly improve the thermal resilience of an existing building. Many of the recommendations provided on these checklists for new homes are also applicable to existing homes. In addition, there are many energy efficiency and durability measures described in the Solution Center’s retrofit list that can increase the winter weather resistance of older homes. Those can be found here:
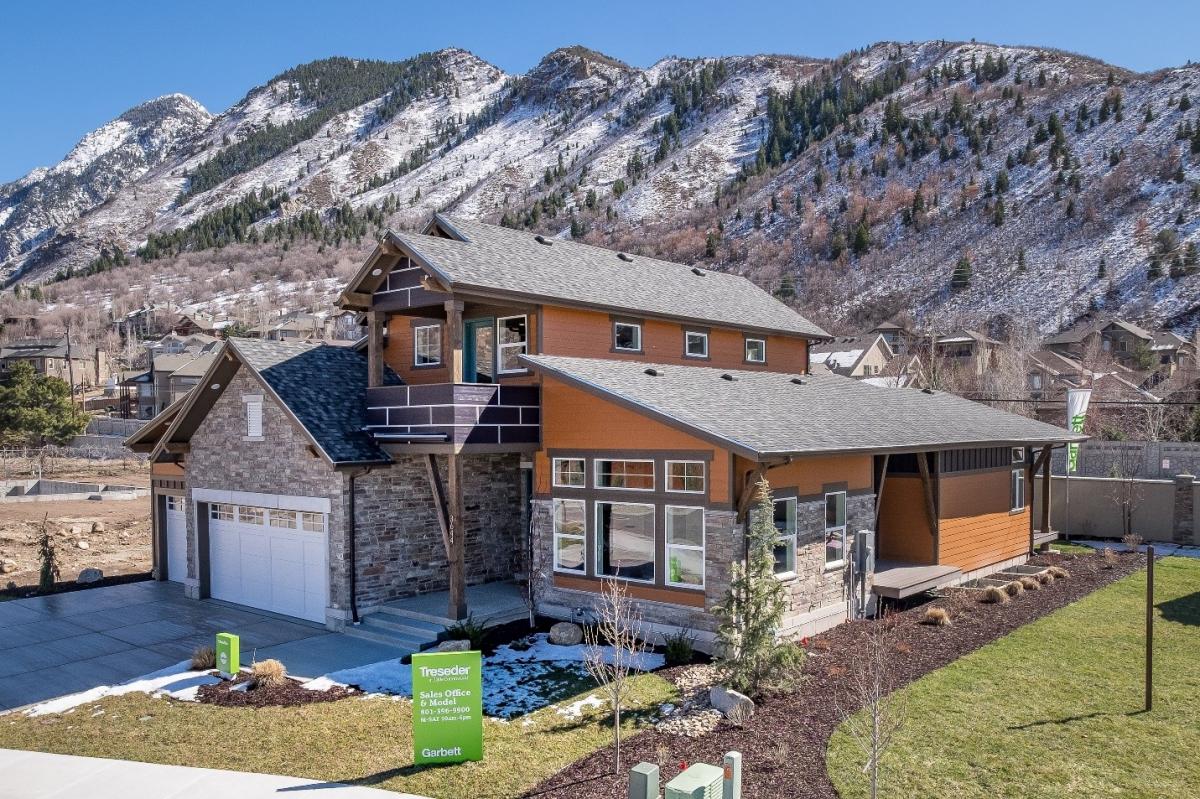
Planning for Severe Winter Weather Events
There are several measures that can be added to a home during or after construction to help protect it from the effects of severe winter weather events. Some of those measures, including back-up generators, secondary heat sources, cold-climate heat pump considerations, plumbing freeze protection, heat tape, venting concerns, and design for thermal efficiency are described below. Many of these and other measures are described in more detail in individual guides linked at the end of this information guide and from the Winter Weather checklist in the Solution Center.
Backup Power Generators
When severe winter weather events interrupt or shut down the electric utility grid, backup power using a portable generator is a relatively affordable resiliency strategy. Generators burn propane, gasoline, or diesel fuel and give off carbon monoxide. They should never be operated inside the home, the garage, or a basement. They should be operated outdoors at least twenty feet away from the home, ideally set on a concrete pad elevated above expected snow levels and protected from rain and falling snow by a roofed enclosure with open or vented sides. In rural locations or where more frequent power outages are anticipated, homeowners may choose to install a larger generator and hook it up to the home’s natural gas line or propane tank. Portable generators would typically be set up to run just a few critical circuits like HVAC, refrigeration, and some plug loads. An electrician is usually required to add a panel or switch to the home’s main electric panel for connecting the generator. See the guide Generators for Back-Up Power During Outages for more information.
Renewables and Battery Storage
If the home has renewable power systems like solar panels or wind turbines, these systems can provide intermittent power to the home when the sun is shining or the wind is blowing, if the electrical system has been set up so that it can be disconnected from the grid and connected to the home’s electric panel or a battery during power outages. A 10-kilowatt battery alone will be able to cover some but not all of an average household’s electric needs for about one day before needing recharging. A battery connected to a renewable system like PV panels may be able to meet all of a home's power needs on most days, depending on the size of the system and weather conditions. See the guide PV Battery Storage for Power Outages for more.
Secondary Heat Sources
Depending on the climate zone of the building, a secondary heat source is perhaps the easiest way to increase resiliency for severe winter weather events. In climate zones 4 and 5, a secondary heat source would only be used in an emergency but may still be worth the investment. In climate zones 6 through 8, a secondary heat source will be useful not only in emergencies, but in normal winter operations during extended periods of cold. In climate zones 7 through 9, where winter temperatures can become dangerously cold, it is an unnecessary risk for a home to depend on only one heat source. The secondary heat source for the building should not require the same fuel type as the primary heat source. In rural areas, a sealed wood-burning appliance can provide heat during severe winter weather events without the need for electricity. Several models of wood stoves and masonry heaters are ENERGY STAR labeled. Other options for secondary heat sources are non-electric pellet stoves and direct-vent non-electric gas heaters. Chimneys should be inspected and cleaned annually. Older fireplaces can be fitted with efficient sealed-combustion inserts that will improve safety and performance. See the guide Fireplaces and Wood Stoves Have Proper Ventilation for more in formation.
Heat Pumps for Cold Climates
Heat pumps for space conditioning are becoming more popular in cold climates. Aftermarket heat trace can be installed on the outdoor unit. The heat trace can be wired to a glowing electric pilot switch in the mechanical room that will light up to indicate when the heat trace has been switched on. Turning on the heat trace only during times or conditions when needed is preferable to supplying the heat trace with hard-wired continuous power. In climate zones 4 and up, a cold-climate-specific heat pump can be installed, which is equipped with a built-in defrost cycle and has a greater capacity at colder temperatures. See the guide Traditional Split Heat Pumps.
Plumbing Resiliency Measures
Frozen pipes are a common and very expensive problem during periods of uncommonly low temperatures or power outages during winter events. The following checklist outlines design and retrofit measures that may save a home from expensive damage:
- Ensure shutoff valves to outdoor faucets and the main shutoff valve for the home or the power shutoff for the pump are installed to be accessible from indoors.
- Install bleeder caps on shutoff valves.
- Install frost-free sill cocks, with the pipe to the sill cock sloped at a slight downward pitch.
- Apply insulating hose bib covers during the winter.
- Where possible, do not place drains back to the main on exterior walls.
- Provide the ability to drain the water lines for homeowners who will be leaving the home for extended periods during the winter.
- Use cross-linked polyethylene (PEX) piping, which has proven to be more robust to freeze-thaw cycling than traditional copper piping.
In homes where the plumbing runs are in a crawlspace, the following additional protection measures should be considered:
- Seal all penetrations through rim joists and walls with expandable, closed-cell foam.
- Insulate piping runs.
- Apply heat trace to piping runs (see next section).
See the guide Insulating Plumbing Pipes.
Heat Trace/Tape for Freeze Prevention
Heat trace or tape is electric resistance cable that can be applied to plumbing lines, drain lines, and vent stacks in cold climates to prevent freezing. Heat trace should be on its own circuit as a best practice and, in order to save energy when it is not needed, it should be switched to a glowing pilot switch in a mechanical room that is labeled for occupants. Heat trace can also incorporate its own thermostat to self-regulate its operation. In regions where cold events are rare, aftermarket heat tape that can be plugged in with an extension cord may be sufficient. Plug-in heat tape should only be plugged in to a GFCI receptacle. In climate zones 6-8, dedicated heat tape hardwired to the electrical panel by a licensed electrician is more robust, safer, and less likely to be influenced by human error. See the guide Insulating Plumbing Pipes.
Venting
In incidences of unusually high snowfall, the snow depth on the roof may exceed the height of the plumbing vents. Additionally, extended periods of cold weather can cause plumbing vents through the roof to ice up and freeze. In both of these incidences, sewage gases may be unable to vent, which can cause odors and air quality problems within the home. These problems are more likely to occur with well-insulated roofs in very cold climates, where there may not be enough heat leaking through the thermal envelope to keep the vent from freezing up. Heat trace can be installed on plumbing vent-to-roof connections and switched to a pilot light switch in the bathroom, so that the occupant can turn on the heat trace to melt the ice when sewer gases are detected in the home as a result of a frozen or snow-covered vent. It is also possible to increase the vent diameter above the uninsulated roof cavity to allow space for gas to exit the vent pipe without causing icing. Check local code before using this strategy.
Winter Weather Guides
The following Building America Solution Center guides provide information on making the components and assemblies of a home more resistant to severe winter weather.
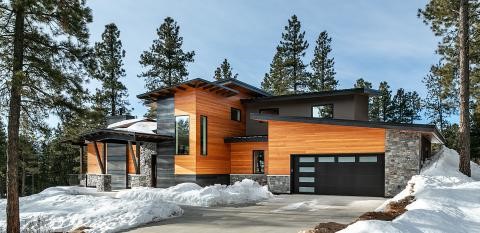
Roof Types
- Asphalt Shingle Roofs
- Metal Roofs
- Clay or Concrete Tile Roofs
- Managing Snow Loads on Roofs and Decks
Roof Sealing, Sheathing, Venting
- Condensation Control in Attics and Roofs in Cold Weather
- Roof Valleys and Penetrations Sealed
- Roof Eaves Sealed with Self-Adhering Membrane in Cold Climates
- Construct Roofs and Attics for Ice Dam Prevention
Roof Attachments:
Attics:
- Attic Air Sealing, Insulating, and Ventilating for Ice Dam Prevention
- Attic Eave Minimum Insulation
- Unvented Attic Insulation
- Air Sealing Attic Access Panels/Doors/Stairs
- Attic Knee Walls
- Air Seal Top Plates or Blocking Missing at Top of Walls Adjoining Unconditioned Spaces
- Air Sealing Drywall to Top Plate
- Insulating and Air Sealing Existing Non-ICAT Recessed Lights
Walls/Windows/Doors:
- Condensation Control for Walls in Cold Weather
- ENERGY STAR Windows
- Interior Storm Windows and Panels
- Low-E Exterior Storm Windows
- Exterior Doors Are Insulated, Impact Rated and Fire Rated
Building Attachments:
Foundation/Site:
- Air Sealed, Insulated Basements
- Unvented, Insulated Crawlspaces
- Damp-Proof Exterior Surface of Below-Grade Walls
- Slab Edge Insulation
- Carpet Not Installed in Wet Areas
- Final Grade Slopes Away from Foundation
Operations and Equipment:
- Fireplaces and Wood Stoves Have Proper Ventilation
- Generators for Back-Up Power During Outages
- PV Battery Storage for Power Outages
- Managing Indoor Humidity and Ventilation in Cold Weather
- Emergency Supplies Storage
- Insulating Plumbing Pipes
- Combustion Furnaces
- Condensing Boilers
- Gas-Fired Tankless Water Heaters
- Integrated Heating and Hot Water with Tankless Gas or Electric Water Heating
- Gas Fired Boilers
- Direct Vent Equipment
In addition to these guides, the Solution Center contains an extensive collection of references, training videos, images, and webinars on disaster-resistant construction related to winter weather. These can be found by searching “winter weather” in the Solution Center.
The following authors and organizations contributed to the content in this Guide.
National Renewable Energy Laboratory Cold Climate Housing Research Center
Pacific Northwest National Laboratory