Scope
Implement passive and low-energy cooling techniques to reduce or eliminate the use of air-conditioning and to mitigate overheating during power outages.
- Site the house to minimize east/west solar exposure and to maximize landscape shading and water features.
- Build or retrofit the house to minimize heat gain, following the recommendations in the Design for Extreme Heat guide.
- Determine your goals for passive and low energy cooling:
- To act as the primary or only cooling for the house,
- To supplement mechanical A/C as an energy conservation approach, and/or
- To serve as emergency backup cooling during a power outage.
- Determine your IECC climate zone.
- Implement passive and low-energy cooling strategies appropriate for your location, as recommended on the Climate tab of this guide for each climate region.
See the Compliance Tab for related codes and standards requirements, and criteria to meet national programs such as DOE’s Zero Energy Ready Home program, ENERGY STAR Single-Family New Homes, and Indoor airPLUS.
Description
This guide provides practical information on techniques for cooling a house passively or with very low energy usage (Figure 1). See the Design for Extreme Heat guide for strategies to minimize heat gain in a house and for background information on passive and low-energy cooling approaches.
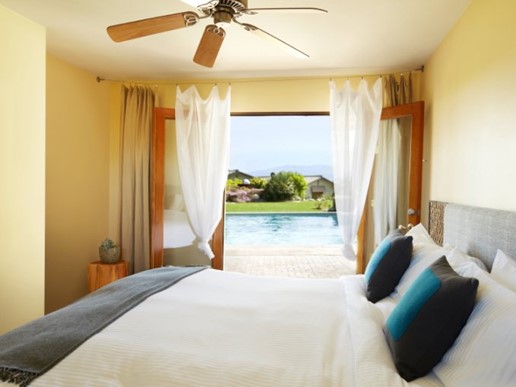
Passive and low-energy cooling includes any cooling approach that uses significantly less energy than a standard air-conditioning system. Some approaches use no energy at all. The main sources of low-energy cooling are cool outside air, evaporation, the ground, thermal radiation to the sky, and waste cooling from appliances such as heat pump water heaters.
Passive and low-energy cooling can be incorporated into a home’s overall cooling approach by:
- acting as the primary source of cooling for homes designed without mechanical air-conditioning,
- replacing the use of air-conditioning during part of the cooling season to reduce energy consumption and HVAC equipment wear,
- operating in tandem with mechanical air-conditioning to reduce the load on the systems, and/or
- acting as a backup cooling strategy during power-outages.
Local climate can strongly impact the effectiveness of various methods. Dry climates have more passive cooling options than humid climates, and cooler climates have more opportunity than hotter climates. See the Climate tab for specific recommendations for each climate region.
These strategies can be highly effective. However, they should be implemented with realistic expectations. Put plainly, passive and low-energy cooling approaches are rarely as effective, versatile, and controllable as mechanical air-conditioning. The expectation that a person can simply set a thermostat to a comfortable temperature and expect a passive system to maintain that temperature during all types of weather is almost always unrealistic. Further, few if any passive and low-energy systems can compete with mechanical systems in terms of providing precise and effective dehumidification.
Minimizing Heat Gain
The first step in any cooling strategy should be to minimize heat gain during the cooling season. See the Design for Extreme Heat information guide for a comprehensive list of strategies to minimize heat gain. Focus on these strategies first before implementing passive cooling strategies. It is generally easier to block heat from entering a house than to remove it once it is there (Figure 2).
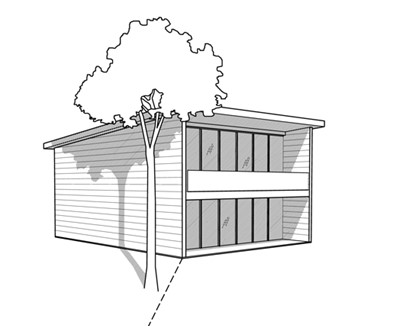
Heat enters a house by solar radiation directly through windows and skylights, by conduction through roofs, windows, and walls, by intentional mechanical ventilation, and by infiltration of hot outside air through gaps and leaks in and around windows, doors, walls, roofs, and floors. Internal heat gains come primarily from cooking, showers, large appliances, and people themselves, but even efficient lighting and small appliances will contribute (Figure 3).
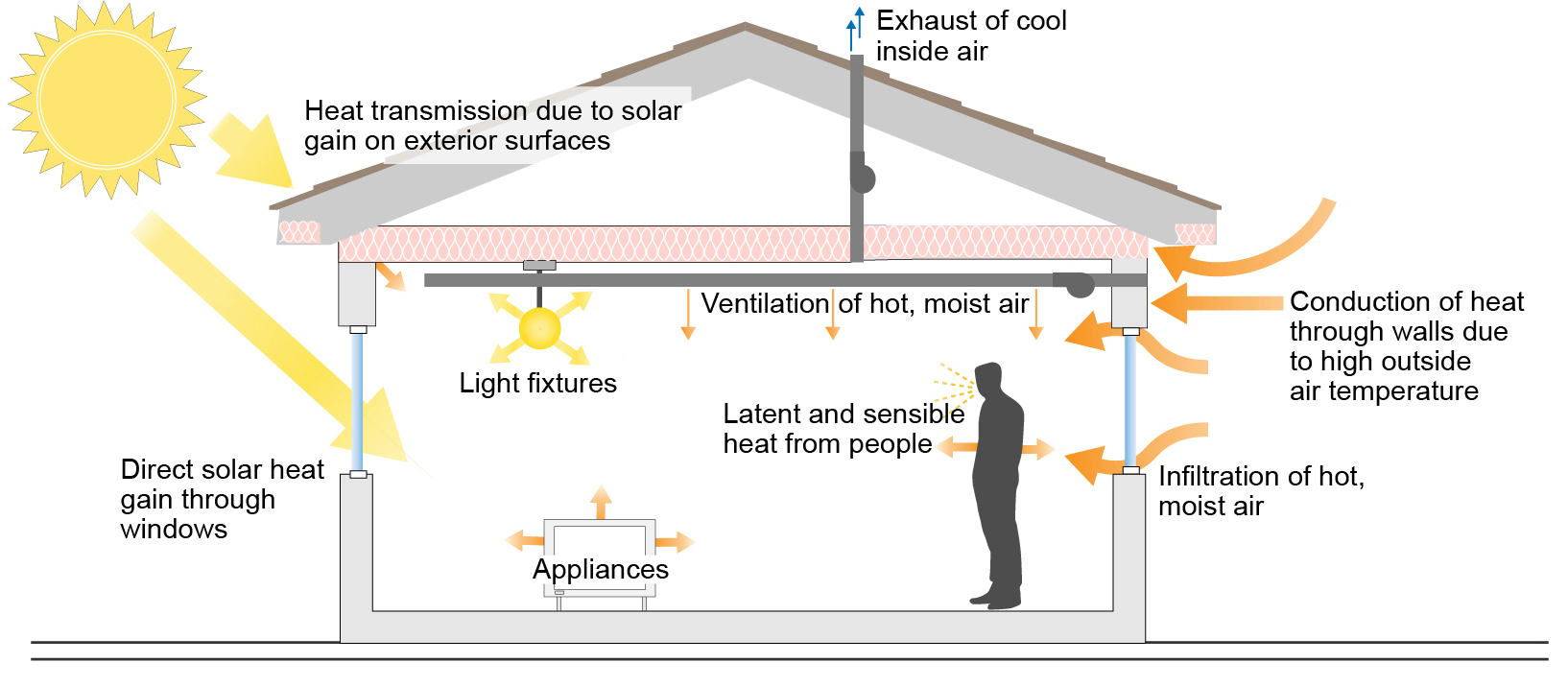
Minimizing heat gain begins with providing a high-performance envelope including well-insulated roofs and walls, minimized thermal bridging, low solar heat gain coefficient (SHGC) windows, and tight air-sealing. Simply following the local building energy code will often go far toward achieving a high-performance envelope, but adhering to stricter requirements such as those for the U.S. Department of Energy (DOE) Zero-Energy Ready Home (ZERH) program will greatly improve performance. See the Air Sealing, Insulating, and Venting Attics and Roofs to Reduce Heat Gain guide, the Air Sealing and Insulating Walls to Reduce Heat Gain guide, and the High-Performance Windows guide for more information.
Effective shading and solar control are highly important. Solar control strategies are usually the most impactful measures one can take to minimize heat gain. Shading and solar control should prioritize skylights and windows first, the roof next (if feasible), and the walls last. East- and west-facing windows and walls should take priority over north- and south-facing windows and walls. Effective shading can block direct sun while allowing daylight in to reduce the use of electric lighting. Solar control measures include low SHGC window coatings, high solar reflectance roof and wall surfaces, and shading devices such as overhangs, manual or automated exterior solar screens, and permanent or seasonal awnings (Figures 4, 5, and 6). See the Shading and Solar Control for Windows and Skylights , Window Attachments for Solar Control and Energy Efficiency, and the Cool Roofs and Walls guides for more information. See the Cool Roof Rating Council website for product directories listing Solar Reflectance Index (SRI).
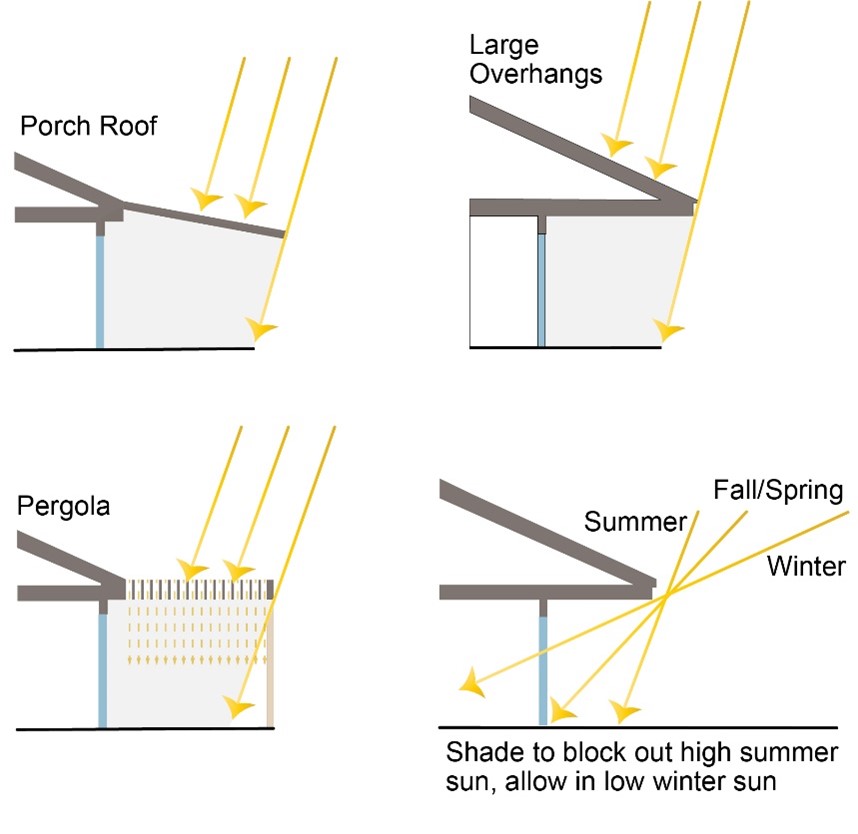
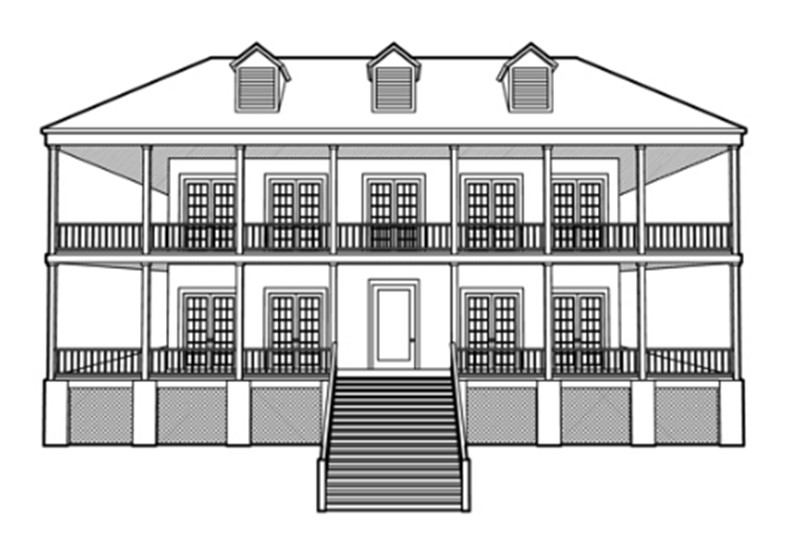
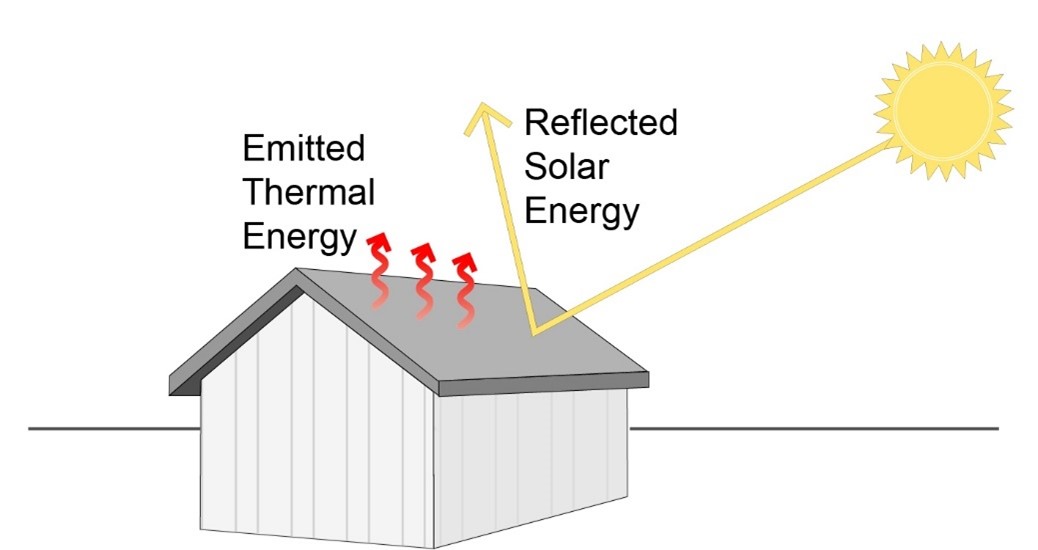
Internal gains can be a significant heat source in a home. Specifying high-efficiency appliances and lighting and providing bath and kitchen exhaust fans to remove heat and humidity from showers and cooking will help reduce internal heat gains. Encouraging homeowners to turn off all unnecessary plug-in equipment and use power strips with energy-saving auto shut-off features will further minimize internally generated heat.
Ventilation air, introduced by the HVAC system, exhaust fans, or supply fans, will contribute significantly to heat gains during hot and/or humid weather. Avoid over-ventilating the house to minimize heat gain through the introduction of outside air. ASHRAE standard 62.2 provides guidance on ventilation rates for homes. Incorporating energy recovery ventilation can greatly reduce heat gains to an air-conditioned home.
Landscaping is one of the most valuable tools available for minimizing heat gain. Design landscaping to maximize shading and evaporation while minimizing solar heat absorption (Figure 7). Vegetation, water features, light-colored surfaces, and water-permeable surfaces are helpful, while pavement, hard scaping, dark surfaces, and water-impermeable surfaces are detrimental. See the Landscaping to Reduce Cooling Load guide for more information.
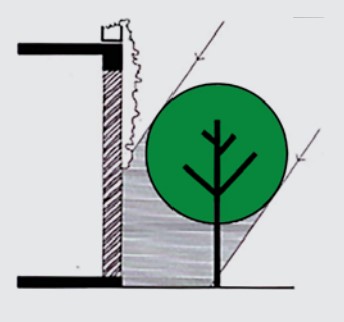
Note that some of these strategies will increase heating loads in the winter. These strategies should be carefully considered in cooler climates. For example, extra low SHGC windows are likely not appropriate on south-facing windows in cold climates. Cool roofs will similarly increase heating needs in winter. A home energy rater can help evaluate the possible impact of different improvements on annual heating and cooling energy use.
In summary, the primary steps to reduce heat gains to a home are as follows:
- Provide a well-insulated and air-sealed envelope.
- Provide effective shading and solar control.
- Reduce internal gains.
- Avoid over-ventilating the house and incorporate energy recovery ventilation.
- Design landscaping to maximize shading, water retention, and evaporation.
Siting, Orienting, and Shaping the House
The first opportunity to minimize heat gain and maximize passive cooling potential in new construction is in siting, orienting, and shaping the house. Perhaps the most important consideration is how the sun interacts with the home. The sun’s path across the sky changes throughout the year. In the summer, the amount of solar radiation striking the roof and the east- and west-facing walls increases significantly as compared to the north- and south-facing walls (See Figure 8).
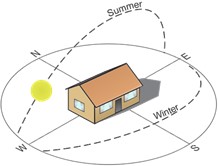
The amount of time that the summer sun shines more directly on E/W walls than on N/S walls is dramatic at most latitudes. In the middle of the summer, unshaded E/W walls receive about two times more solar heat per square foot than unshaded N/S walls at the latitudes of the contiguous United States. This effect is more significant at more southerly latitudes.
The path of the sun in the summer months greatly increases solar radiation on the roof as well. One square foot of roof area will receive approximately 3 to 4 times as much solar radiation on a midsummer day as one square foot of north- and south-facing walls. See Table 1.
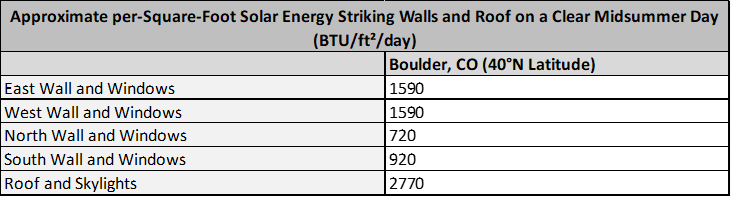
Elongating and orienting a house on an east/west axis will minimize solar heat gain. This orientation will decrease wall and window area to the east and west, where solar gains are greatest in the summer. Note that this strategy is ideal for maximizing passive solar heat gain in the winter as well as minimizing solar heat gain in the summer. See Figure 8 above and Figure 9 below.
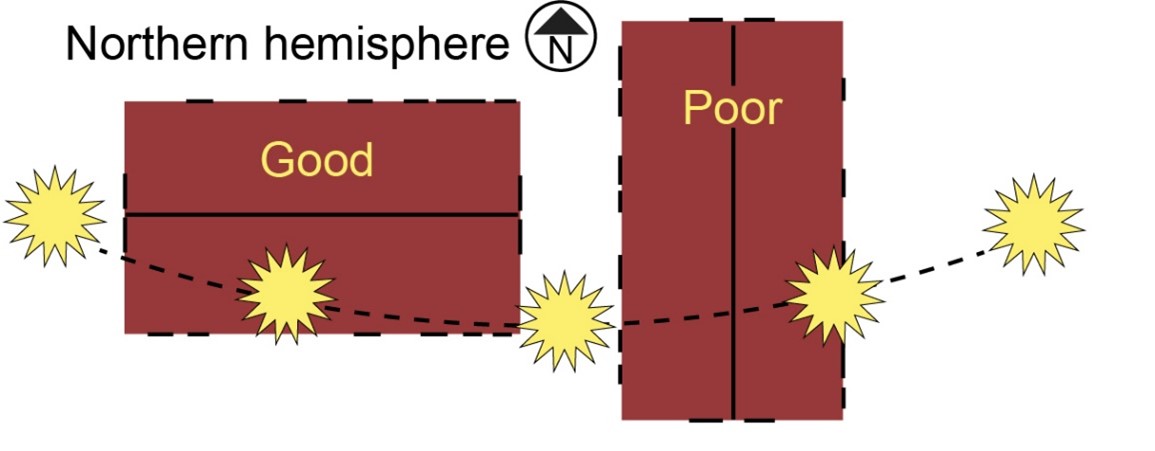
Regardless of house shape or orientation, limiting the total amount of window and glass door area will have a significant effect on overall heat gain. Windows and glass doors should especially be minimized on the east and west.
More compact construction will result in less roof and wall area per square foot of floor area, reducing overall heat gain. Creating a more compact roof and wall envelope may conflict with the concept of elongating the house on an east/west axis, however. If an east/west orientation is not possible or desired, using more compact construction should be considered.
Building up rather than out offers several advantages from a passive cooling standpoint. Changing a house design from one story to two stories can reduce roof area, which reduces summertime solar heat gain. A two-story house also allows stratification which will create a cooler zone in the lower portion of the house. A second story further allows for upper windows to take advantage of the greater velocity wind found higher from the ground. Also, vertical height allows for natural stack effect to help exhaust heat.
Non-conditioned spaces, such as a garage, can be located on the east or west side of the house as a strategy to reduce heat gains to the conditioned areas. Locating a non-conditioned space on the east or west will allow it to act as a buffer zone between the conditioned space and the side of the house with the greatest solar load.
A house should be sited to best take advantage of local water features, existing trees, structures, or land features as sources of shade and evaporative cooling (Figure 10). Prioritize shading of the roof and the E/W walls. See the Landscaping to Reduce Cooling Load guide for more information.
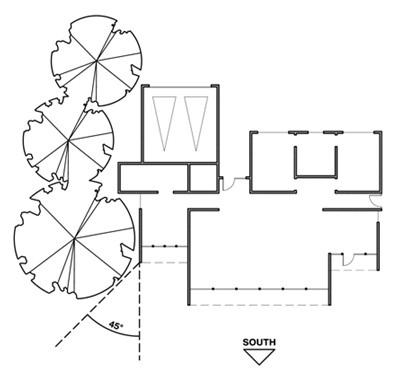
Wind is an extremely valuable resource for passive cooling, but there is generally no need to make great efforts to site the house to take advantage of local wind patterns. In most locations in the United States the wind direction is variable. Even in areas with very predominant summer winds, there is not much value in attempting to face the home directly into the wind. A house can be oriented as much as 60 degrees off from the predominant wind direction and still use the wind as effectively or more effectively than a home oriented to face the wind directly (Givoni 1991) . Siting to optimize shading and solar control should take priority over wind direction.
Wind velocities tend to increase significantly with elevation above ground level (see Figure 11). Building on higher ground or designing a taller house can take advantage of this effect for natural ventilation. However, this may not be advisable in a heating-dominated climate where winter wind will increase heating energy consumption.
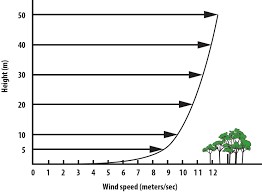
Land features, adjacent buildings, and vegetation can block, filter, funnel, and alter the direction and intensity of winds. In general, siting a home too closely to upwind or downwind buildings or tall land and vegetation features will reduce the available wind for natural cooling. However, the shading benefit provided by such features can easily outweigh any reduction in wind speeds that they cause.
In summary, when siting, orienting, and shaping a house, consider the following recommendations:
- Orient the house on an E/W axis.
- Minimize window area, particularly on the east and west.
- Minimize roof and wall area (compact construction).
- Consider building up rather than out.
- Plan for non-conditioned spaces to be on the east or west side of the house.
- Site the house to best take advantage of local water features, existing trees, structures, or land features as sources of shade and evaporative cooling.
- Consider building on higher ground for improved natural ventilation.
Airflow and Ventilation Cooling
Passive cooling strategies using airflow are perhaps the most widely applicable, cost-effective, and simple passive measures available. They can be divided into two separate strategies: Comfort ventilation and exhaust cooling. The exhaust cooling strategy can be refined into a sub-strategy called night flush. Implementation of these strategies can overlap significantly, and sometimes implementing one will automatically implement another.
Comfort Ventilation
Comfort ventilation is a well-known person-focused cooling strategy. As air moves across a person’s skin, it speeds heat loss through evaporation, the body’s primary natural cooling mechanism. Studies show that people can remain comfortable in higher ambient temperatures when the air speed around their bodies is increased. Comfort ventilation does not actually lower the air temperature, but the cooling effect on a person’s body is real. It has been shown that when using ceiling fans at the same time as air-conditioning, the thermostat can be turned up by about 4°F (Cook and Chandra 1989).
Figure 12 shows the cooling effect a person feels when exposed to increased air speeds (based on results derived from the CBE Thermal Comfort Tool 2.4.2 (ASHRAE-55)). The figure indicates that an air speed of 150 feet per minute (FPM) will make an occupant feel approximately 5°F cooler. If the actual temperature inside the room is 82°F, this means the person will feel as though the air is about 77°F. The effect would be greater at lower humidity.
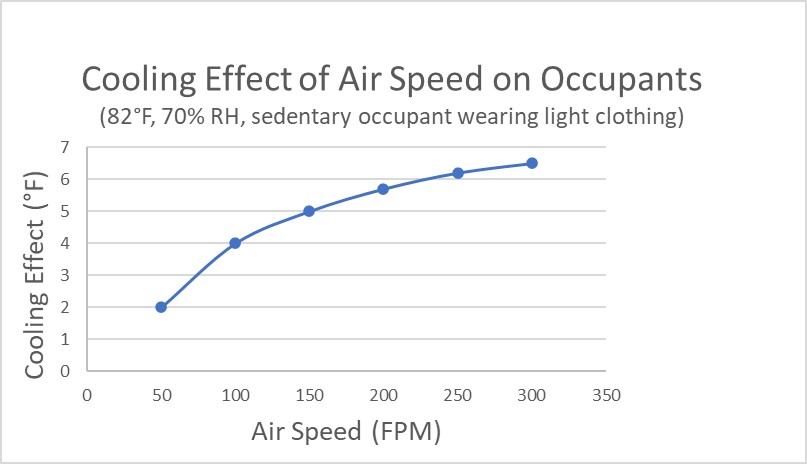
The air speeds shown are very achievable for a wind-ventilated room or a room with a ceiling fan. Note that at 160 FPM papers may rustle, and some occupants may not enjoy speeds above 300 FPM. In practice, a reasonable rule of thumb is that comfort conditions can be met without A/C using comfort ventilation at temperatures up to about 82°F (Abrams 1986). If other options aren’t available, however, comfort ventilation can be used to provide some level of cooling at ambient temperatures well above 82°F.
There is an upper limit of temperature and humidity when airflow across the skin will provide no cooling effect or will even have a detrimental effect. An ambient temperature of 95°F is often cited as the upper limit for temperature (Steadman 1979). However, more recent studies indicate a healthy adult can still experience a net cooling effect at temperatures in the 104-108°F range, at 50% humidity (Morris, et al. 2021). At such temperatures, however, no amount of airflow can be expected to provide standard comfort conditions.
Because comfort ventilation cools people directly, air should be directed to flow across the skin of occupants (Figure 13). The primary sources of airflow available for comfort ventilation are wind, ceiling fans, portable fans, and whole-house fans. Of these, ceiling fans are the most widely applicable and reliable building-integrated option as they can be used in any climate with or without windows open, with or without available wind, and with or without air-conditioning.
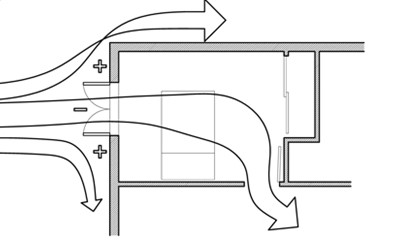
The keys to achieving comfort through comfort ventilation include:
- ambient temperatures below about 82°F
- air directed to flow across occupants
- interior air speeds between 100 FPM and 300 FPM
- effective shading.
Exhaust Cooling
The exhaust cooling strategy is simple and effective: when the indoor air is warmer and more humid than the outdoor air, exhaust it. Exhaust cooling can be used in any climate and can work in conjunction with almost any other passive cooling strategy. In humid environments, however, this strategy can introduce high-humidity air which may be uncomfortable. Other than kitchen and bath exhaust fans, this strategy should generally not be used in coordination with air-conditioning in humid climates, as high-humidity outdoor air could increase energy consumption of air-conditioning systems even if the outside temperature is lower than the inside temperature. See the Whole-House Fans guide for guidance on whole-house exhaust cooling strategies in humid climates.
Exhaust cooling is most effective when air is exhausted from the hottest parts of the house (Figure 14). These include the upper-floor ceiling peaks, skylight wells, over the kitchen range, and in bathrooms with showers. Operable skylights, whole-house fans, range hoods, and bathroom fans all take advantage of this principle.
When indoor air is exhausted, cooler outdoor air will replace it. The intake air should come from multiple window or vent locations around the house so that air flows through all rooms. Ideally the intake locations should be well-shaded.
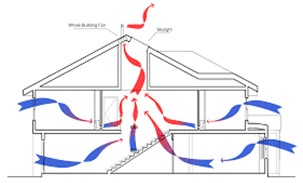
Unlike comfort ventilation, exhaust cooling actually removes heat from the house. For example, consider a house that utilizes steady wind or a whole-house fan for exhaust cooling. In this scenario the exhaust airflow could easily be around 5,000 CFM or more. If the temperature outside is 72°F, the temperature inside is 78°F, and the humidity outside is similar to the humidity inside, the cooling provided by 5,000 CFM is calculated to be about 33,000 BTU/hr, which is about the same amount of cooling provided by a medium-size centrally ducted air conditioner. If there are sources of humidity inside the house, the calculated exhaust cooling would be greater.
Lower airflows would result in less cooling, but can still be effective if the warmest and most humid air in the house is targeted. For instance, a small exhaust fan (such as a bathroom fan) would be a weak contributor to a whole-house exhaust cooling strategy unless it were located near a shower, cooking station, or other source of high heat and humidity. A bathroom exhaust fan, though it may only exhaust around 100 CFM, can prove very valuable due to the hot, high-humidity air it exhausts during and after a shower.
Regardless of how much exhaust airflow a house has, it cannot be cooled lower than the outdoor air temperature. For this reason, exhaust cooling alone cannot be expected to provide comfort unless the outdoor air temperature is around 75°F or lower. It can improve conditions, however, at any temperature as long as it is cooler outside than inside and not excessively humid outside.
There are three situations where exhaust cooling is most useful:
- high solar gains in the space
- high internal heat/humidity sources such as cooking or showers
- cool outside temperatures, such as at nighttime.
The first condition is mostly avoidable by design and operation of the house. It would be better to control solar gains by shading than to have to remove the heat by exhaust cooling. For the second condition (internal heat gains), exhaust cooling is most effective at the source. Localized exhaust cooling using bath and kitchen fans is very effective at dealing with isolated internal heat sources such as cooking and showers. The third condition (cool outside air) should be taken full advantage of using high airflows throughout the house whenever possible (unless outdoor humidity is excessive).
The primary sources of airflow available for exhaust cooling are wind, whole-house fans, bathroom and kitchen exhaust fans, and the stack effect (Figure 15). Ceiling fans will not contribute to exhaust cooling.
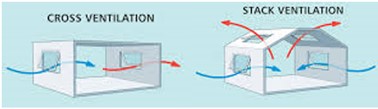
The keys to effective exhaust cooling include:
- outside air that is cooler than inside air
- outside air which is not polluted, smoky, or excessively humid
- airflow volume (CFM) great enough to remove internal and solar heat gains
- exhaust fans placed in the hottest and most humid locations in the house
- intake locations dispersed around the house perimeter
- shaded, protected intakes
- overall effective shading.
Night Flush
Night flush is a form of exhaust cooling. The strategy is to flush the house with cool air at night, then close the house down to keep the coolness inside during the day. Windows, vents, and exhaust fans are open/on during the night and closed/off during the day. Most exhaust cooling strategies will include flushing the house with cool air at night. What distinguishes a full night flush strategy from basic exhaust cooling is that, with night flush, the house is intentionally designed to be capable of maintaining cool temperatures through the entire day.
Basic exhaust cooling can achieve partial night flush in nearly any climate, with any type of construction. Full night flush, however, requires internal thermal mass, high rates of airflow, and a fairly dry climate with cool nights.
A house designed for a full night flush strategy must be able to store and use the coolness of the night to maintain comfortable conditions through the day. This requires thermal mass. Thermal mass is any material that will readily store, conduct, and release heat. Thermal mass in a house can be cooled overnight by nighttime air, allowing it to absorb heat through the day. When thermal mass is absorbing heat, it cools both the space and its occupants. Typical building materials with good thermal mass properties are concrete, masonry, drywall, metal, and phase change materials (PCMs). See the High-Thermal-Mass Construction guide for more information.
There must be ample area of thermal mass to provide enough heat transfer to and from the air. Only the first one or two inches beneath the surface of a wall, ceiling, or floor will contribute meaningfully to a night flush strategy. For example, interior plaster walls will provide large areas of useful thermal mass. In comparison, a thick concrete column will not be as useful because it has very little exposed area; much of its mass is located too deep in the core of the column.
The thermal mass must be exposed to the inside air. It must also be on the inside of the home’s insulation layer. For example, a stained and sealed concrete floor exposed to the inside would provide excellent thermal mass (Figure 16). If the concrete were covered in carpet, however, it would be too insulated from the inside air to be able to provide much benefit for a night flush strategy.
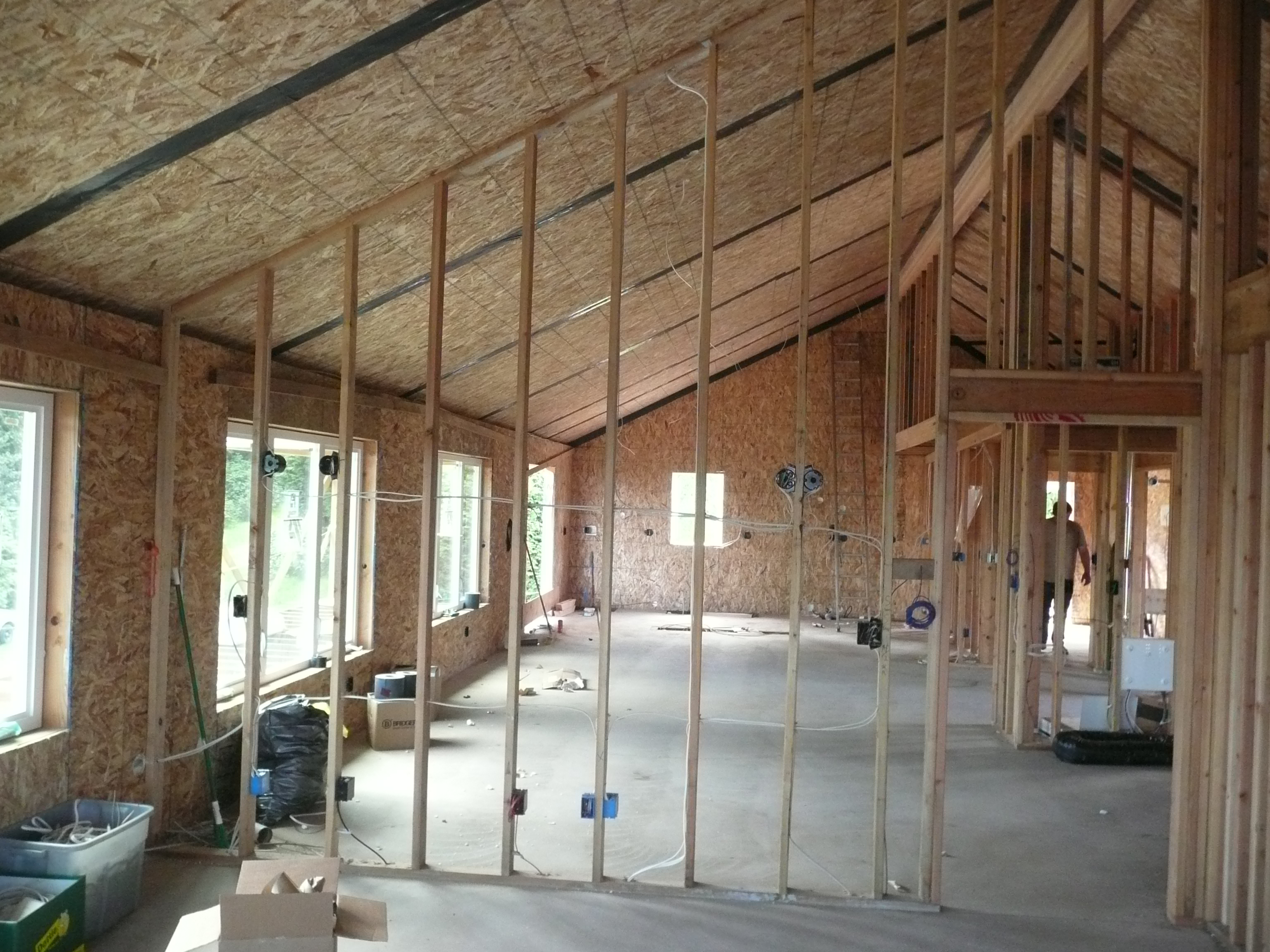
Incorporating thermal mass does not require masonry construction instead of wood framing. One way to include large areas of exposed thermal mass is to use thicker and/or double-layered drywall on walls and ceilings. Stucco, tile, or plaster walls, and exposed slab or tile floors are also effective ways to increase thermal mass. Kitchen and bathroom countertops and islands made of granite, soapstone, concrete, tile, stainless steel, quartz, or marble will further contribute to the thermal mass of a home. All of these approaches can be used with wood-framed construction.
While a comfort ventilation strategy directs air to flow across the occupants, a night flush strategy should try to flow the air across the interior thermal mass. This allows the internal components of the house to cool down more quickly and thoroughly (Figure 17).
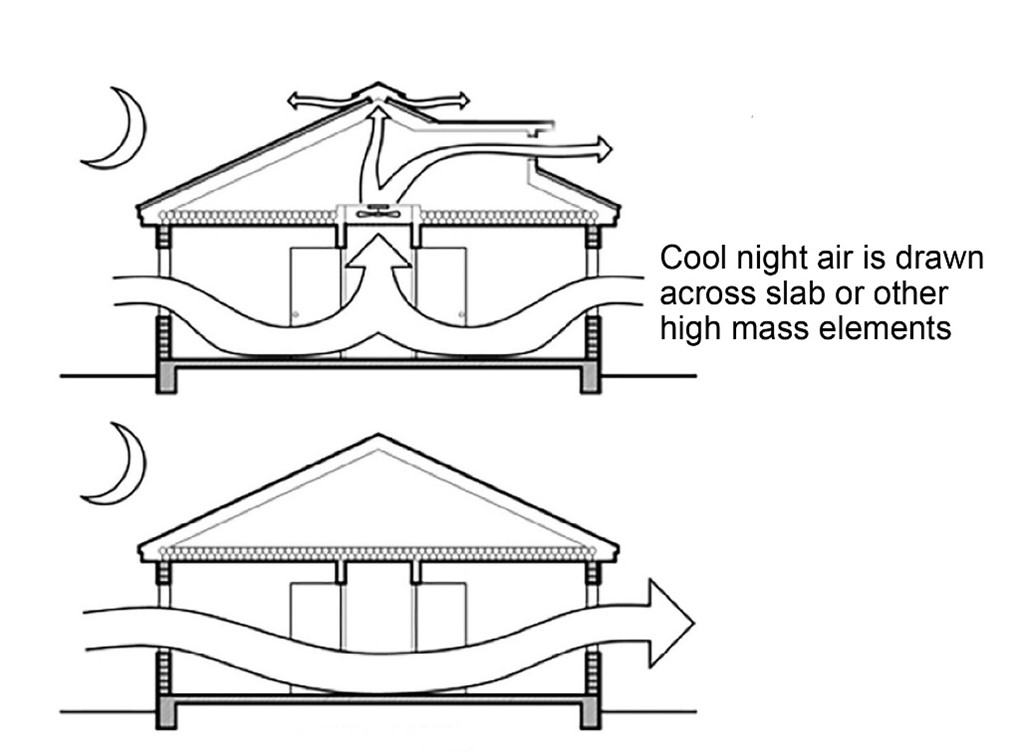
Removing the heat from a home’s thermal mass at night can require a large volume of air entering and leaving the house. The amount of air required for a full night flush strategy depends on climate, house size, the area of exposed thermal mass, how well the air flows across the thermal mass, heat gains during the day, and other factors. Recent recommendations generally fall in the range of about 12 to 23 air changes per hour (ACH) (DOE 2012, CEC Title 24 2019, FBC 2020, PG&E 2004 ). This is equivalent to about 0.2 to 0.375 CFM per cubic foot of conditioned space (floor area x ceiling height). As an example, 20 ACH in a 2,000-square-foot home with 8-ft ceilings equals about 5,300 CFM.
A full night flush strategy generally requires a climate where nighttime temperatures in the summer stay below about 67°F and summer diurnal temperature swings are greater than about 25°F (Givoni 1991). The greater the day-to-night temperature swings, the more opportunity there is for night flush. Night flush is least effective in humid regions or in urban areas where the heat island effect can cause air temperatures to remain high through much of the night. Investing significant resources in thermal mass for a night flush strategy may not be justified unless the above climate criteria are met and the cooling season is longer than just a few weeks. See the Climate tab as well as the Whole-House Fans guide for more information on where the night flush strategy is most appropriate.
The primary sources of airflow available for night flush are wind and whole-house fans. Whole-house fans are particularly useful for night flush because summertime winds tend to die down at night. The stack effect (described in the next section) can aid night flush when wind or fans are not available.
The keys to achieving comfort through a full night flush strategy include:
- nighttime low temperatures below about 67°F
- day to night temperature swings greater than about 25°F
- large areas of thermal mass exposed to the building interior
- high volume of night air flowing through the house (at least 12 to 23 air changes per hour)
- air directed to flow across interior thermal mass surfaces
- outside air that is not polluted, smoky, or excessively humid
- effective shading
- effective air-sealing and insulation.
Sources of Airflow
Sources of airflow for passive and low-energy cooling include wind, the stack effect, whole-house fans, ceiling fans, and small exhaust fans (such as kitchen and bath fans).
Wind
Natural wind is a plentiful zero-energy source of airflow for comfort ventilation, exhaust cooling, or night flush. Sufficient wind speeds are available in many U.S. locations for much of the summer. Obtaining wind data for your location is a good start to understanding local wind patterns. The Climate Consultant program is a free tool that uses statistical weather data files to provide climate information for hundreds of locations. This tool allows quick assessment of local wind patterns. Be aware, however, that wind patterns at the weather station where data were collected could be significantly different from the wind patterns at a specific house site.
It is not recommended to rely on wind as the sole source of air movement. While plentiful overall, in most areas wind is variable and unreliable. Wind speeds change hourly and are often too weak to achieve sufficient cooling. Wind speeds tend to be lower in the summertime, when they are needed most. Winds tend to slow down further at night, when needed for night flush. In addition, it is not desirable to use wind as a source of airflow if smoke or pollution from wildfires, industry, or other sources is in the air. A sound approach is to apply general concepts to effectively utilize wind in the house while planning for fans as backup.
Wind can be brought into a house through vents, wind scoops, wind towers, or other interesting methods, but operable windows are the most common approach. There are countless ways to arrange building openings and floor plans to promote wind-driven crossflow. Simple, straightforward design decisions about window size, shape, location, and type are usually appropriate, while complex and costly efforts to improve wind-driven ventilation are rarely justified.
To take advantage of wind for passive cooling, locate building openings on both the upwind and the downwind sides of the house (Figure 18). These may be opposite sides of the house, or they may be adjacent sides (e.g. when the wind approaches the house at an angle). In general, simply having operable windows on all sides of the house will work. Windows located only on one side of a house or closed room will promote very little airflow within the home unless modified with wing walls or other wind-guiding elements.
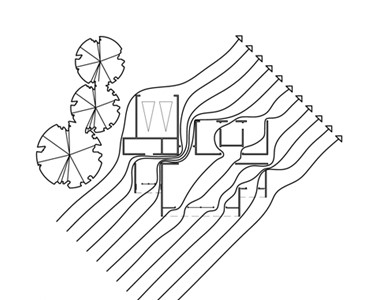
Ensure there is free airflow between upwind and downwind windows (Figure 19). Internal airflow paths can be achieved via open floorplans, transom windows, transfer grilles, jump ducts, or undercut doors (Figure 20). As long as the cross-sectional area of the internal path is at least as large as the air inlets and outlets, it does not need to be direct. It can be offset, convoluted, long, or change levels and still be relatively effective.
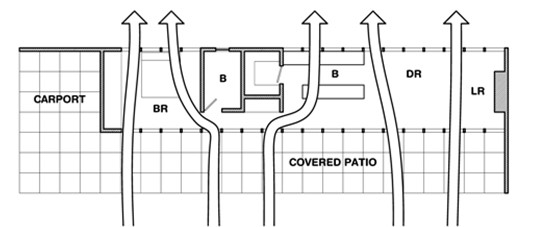
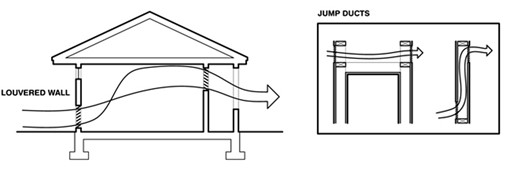
Windows and openings should be easily accessible and convenient to operate. Locate building openings at occupant level if the focus is comfort ventilation (Figure 21). The purpose of comfort ventilation is to increase airflow across occupants’ bodies; locating at least the inlet or outlet windows at occupant level will maximize this effect. If night flush is the focus, locate building openings such that air will flow across the high-thermal-mass elements of the interior (Figure 22).
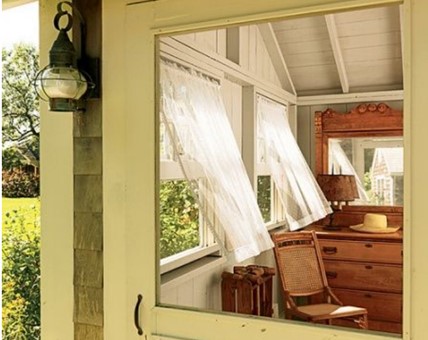
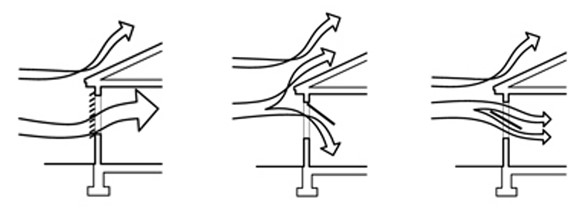
Sufficient inlet and outlet area is necessary for good airflow. Window area is directly related to air flow rate. Greater opening area will allow more airflow. However, there are multiple factors to consider when determining window sizes. One should consider solar gains, thermal losses, view, and architectural aesthetic. Ventilation should be one factor to consider, but not the dominant factor. For instance, it would not be worthwhile to significantly increase the size of east or west-facing windows simply to increase airflow, because solar gains would increase in the summer and thermal losses would increase in the winter.
Casement and awning windows allow airflow through the full area of the window while sliding windows allow airflow through less than half the total window area (Figure 23). Using casement windows instead of sliding windows will more than double your ventilation area without increasing total window area. Insect screens will reduce flow by about 50%. Window treatments and other obstructions can also significantly reduce airflow.
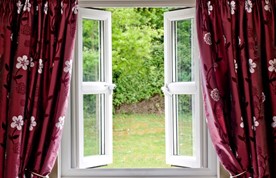
In general, the total inlet area should approximately equal the total outlet area, but this is not of vital importance. Total airflow will be limited by whichever area is smaller. Outlets that are smaller in total area than inlets will reduce inlet air speeds. Outlets that are larger than inlets will increase inlet air speeds.
Wide horizontal windows or inlet openings provide much better flow than tall vertical openings when the wind is approaching at an angle (which is the majority of the time in most locations). When the wind is head-on, the shapes perform equally well (Givoni 1991) .
Windows or other inlets and outlets should be protected from rain using eaves, overhangs, or porches so cross-ventilation can still be accessed during rainy weather (Figure 24). All inlets and outlets should also be well-sealed when closed to reduce energy loss when heating or using air-conditioning.
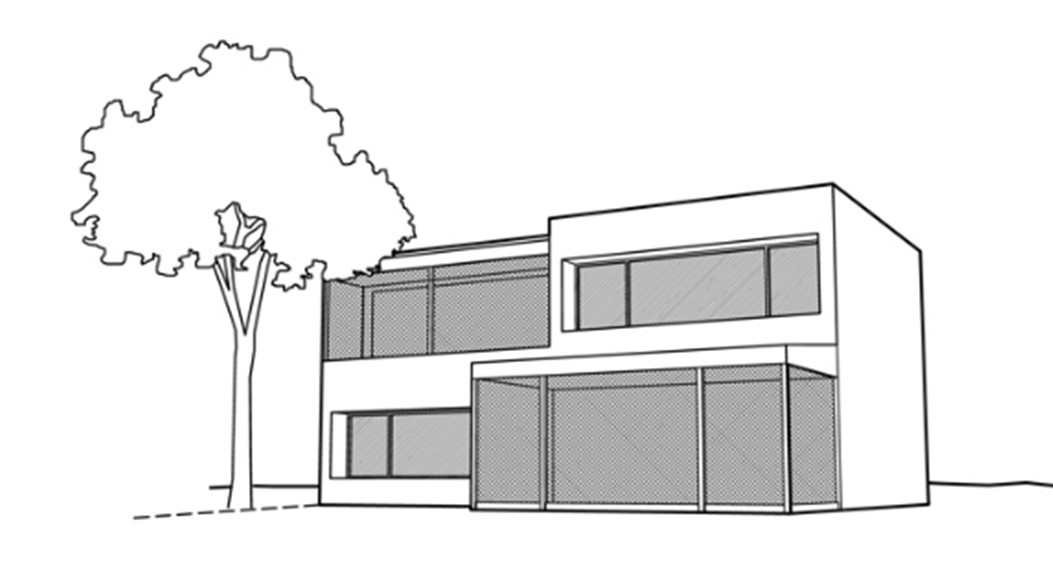
As a general rule, the house should be designed to accept wind from any direction. Wind direction is not constant, even in areas with “prevalent winds.” Most areas of the United States have highly variable wind during the summer, and it is not recommended to take costly measures to design for a predominant wind direction.
However, if the house is in an area with a consistent summertime wind direction such as some coastal and mountain areas, landscape and architectural features can be designed with the wind direction in mind. When cross flow paths in the house do not line up well with predominant wind patterns, architectural features such as fin walls or window fins can guide the wind into and out of the building openings. Landscaping features can have a similar effect.
The basic concept behind any wind-guiding feature is simply that all airflow is caused by a zone of high pressure pushing air to a zone of low pressure. When an obstruction is placed in an air stream, the pressure upwind of the obstruction increases while the pressure downwind of the obstruction decreases. If vegetation, wing walls, or wall fins are placed in an air stream such that a zone of higher pressure is created near a window inlet, then flow into that window will increase. Similarly, if a feature is placed in the air stream such that a zone of lower pressure is created near a window outlet, then flow out of that window will increase. See Figures 25 and 26.
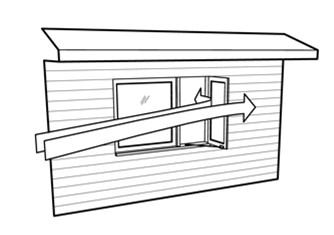
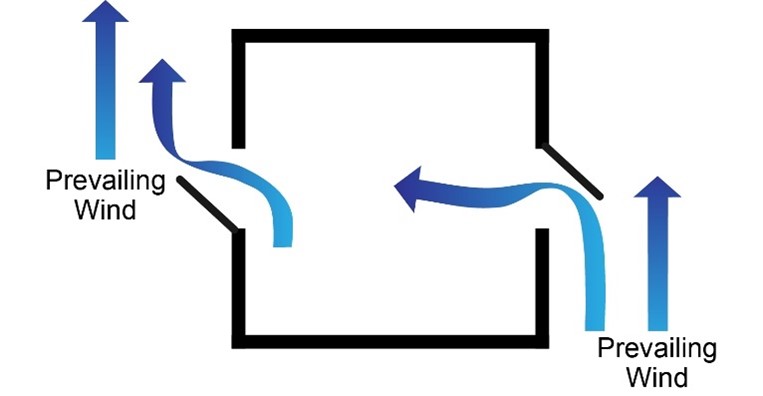
Figure 27 illustrates this concept using vegetation. The schematic on the left shows vegetation which is placed such that it will create a zone of high pressure on one side of the house, while a zone of low pressure is created on the other side of the house. Air is forced to flow through the house. The schematic on the right of Figure 27 shows vegetation which is placed to create high-pressure zones on both sides of the house. These pressure zones work against one another, and cross-ventilation through the house is not induced.
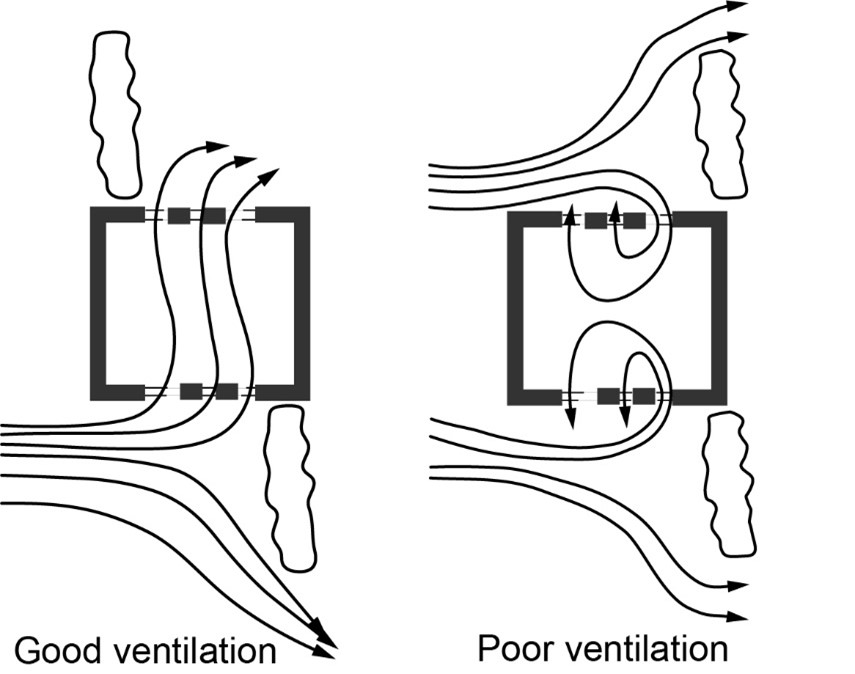
Figure 28 shows various methods of funneling or deflecting wind. Though vegetation is shown in most of these examples, bushes and trees are not nearly as effective as solid features such as wing walls.
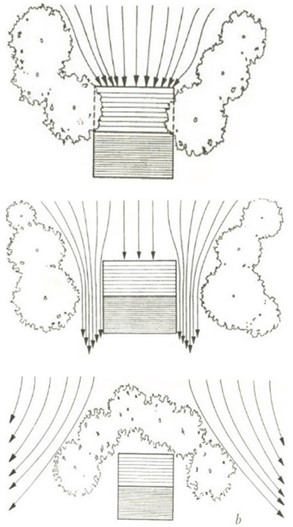
In summary, the recommendations to successfully utilize wind as a source of airflow include the following:
- Locate windows (or openings) on both the upwind and the downwind sides of the house.
- Provide free airflow between upwind and downwind windows.
- Ensure windows and openings are easily accessible and convenient to operate.
- Locate windows at occupant level if the focus is comfort ventilation.
- If night flush is the focus, locate building openings such that air will flow across the high-mass elements of the interior.
- Provide sufficient inlet and outlet area for good airflow.
- Use wide, horizontal window openings rather than tall, vertical window openings.
- Protect inlets and outlets from rain using eaves, overhangs, or porches.
- Ensure all inlets and outlets (windows) are well-sealed when closed.
- Do not rely on wind as the sole source of air movement.
- Design the house to accept wind from any direction.
Stack Effect
The stack effect is a natural mechanism which relies on the fact that warm air is less dense than cool air. Lighter warm air rises while heavier cool air sinks. Using the stack effect intentionally requires air inlets located low in the house and outlets located high in the house. The strength of the stack effect in a house depends in part on the temperature difference between the outside air and the inside air and the height difference between where the air enters and exits the house (Figure 29). At the temperatures and building heights of typical residential construction, the stack effect is a weak force. While it can provide a natural way to exhaust hot air from a building or attic, it is generally not able to produce the air speed necessary for noticeable direct cooling of the body (comfort ventilation).
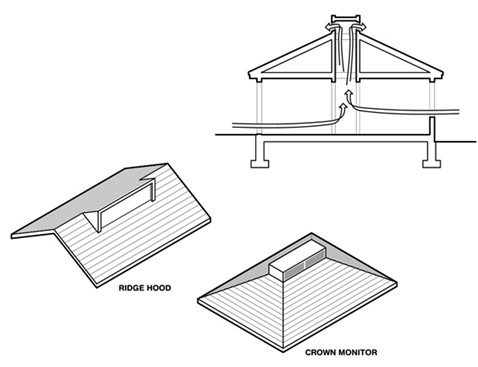
For the stack effect to work at all, the inside of the house must be warmer than the outside. This is counter to the overall goal of cooling, but does coincide well with the exhaust cooling and night flush strategies (which also require inside to be warmer than outside).
An overall large area for both exhaust and intake air is necessary to provide substantial airflow. The total airflow will be limited by the smaller of the total intake area (e.g. low windows) or the total exhaust area (e.g. high windows). A large intake area cannot overcome limitations created by a small exhaust area, and vice versa.
Wind and fans are far more effective at moving air than the stack effect. If no wind is available, and the power is out, however, the stack effect is one of the only options left to create airflow. Creating openings as high and as low in the house as possible is the most straightforward way to maximize the stack effect for night flush or exhaust cooling. Larger openings will allow more airflow.
A solar chimney (a vertical black pipe designed to heat up in sunlight) can increase the stack effect by increasing the stack height above the roof and by creating hotter temperatures inside the stack. With a solar chimney, the inside of the house does not need to be warmer than the outside. However, these devices are designed to work when the sun is shining, which is the opposite of when airflow is needed for night flush. A solar powered fan could also greatly increase exhaust air flows, but these too will only work when the sun is shining and so cannot aid night flush in a meaningful way. The usefulness of these devices is limited for passive cooling of residences.
Overall, stack ventilation is most useful as a passive exhaust mechanism for high-temperature spaces such as an attic.
Consider the following recommendations when incorporating the stack effect for passive cooling:
- Locate air inlets or windows as low in the house as possible.
- Locate air outlets or windows as high in the house as possible.
- Use large (or numerous) windows or vents for both intake and exhaust air.
- Do not rely on stack effect for comfort ventilation.
- Only rely on stack effect as a backup to wind or fans for exhaust cooling or night flush.
Whole-House Fans
Whole-house fans can generate airflow for occupant cooling when wind is not available. The controllability of whole-house fans makes them an attractive backup to wind-driven airflow. Unlike wind-driven airflow, all open windows become air inlets when a whole-house fan is operating (Figure 30).
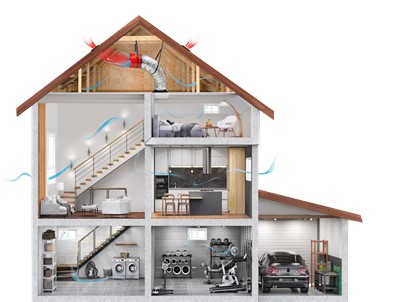
The older traditional design of whole-house fans had a reputation for being noisy and leaky, and required structural modifications to the ceiling and roof system. Newer, smaller ceiling-mounted designs can fit between joists and have insulated dampers. Modern ducted designs use high-efficiency motors, insulated dampers, and noise-dampening features, overcoming most of the concerns related to traditional designs. Most whole-house fans blow air from the house directly into the attic, though there are roof-mounted models available that exhaust directly to the outside. See the Whole-House Fans guide for information on fan designs, sizing and venting criteria, control strategies, and installation recommendations.
Whole-house fans are primarily appropriate for exhaust cooling and night flush, but can contribute to a comfort ventilation strategy as well. Interior air speeds generated by whole-house fans can easily be 100 to 200 FPM near a window, an ideal range to provide cooling of the skin without too much disruption of loose papers or other items. Room-by-room air speeds and comfort can be adjusted by closing some windows while opening others. Overall, however, it is easier to generate comfort ventilation throughout a room using ceiling fans than a whole-house fan.
In non-humid climates, the ideal strategy for operating a whole-house fan would simply be to run it when the outdoor temperature is lower than the indoor temperature or when airflow is desired for comfort ventilation (as long as enough windows are open and the A/C is off). When ideally operated, whole-house fans have shown significant savings in energy models for multiple climate zones (See Table 2).
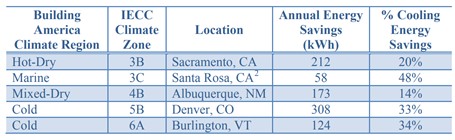
If the home is located in a humid climate, the same strategy can usually be applied as long as air-conditioning is not present or not used. In these regions, however, there may be times when the high humidity of the outdoor air makes it uncomfortable or undesirable even when the outside temperature is lower than the inside temperature. In these situations, it is up to the occupants to decide whether they prefer the fan on or off. It is never recommended to operate a whole-house fan when the outside air temperature is higher than the inside air temperature, as this can cause moisture issues within the home.
Most of the concepts listed for wind-driven ventilation apply to whole-house fan ventilation: locate windows/inlets on multiple sides of the house (Figure 31), provide free flow between air inlets and the whole-house fan, locate windows/openings at occupant level for comfort ventilation or to flow across thermal mass for night flush, ensure windows are accessible and easy to operate, ensure windows are of sufficient size, and protect windows/inlets from rain.
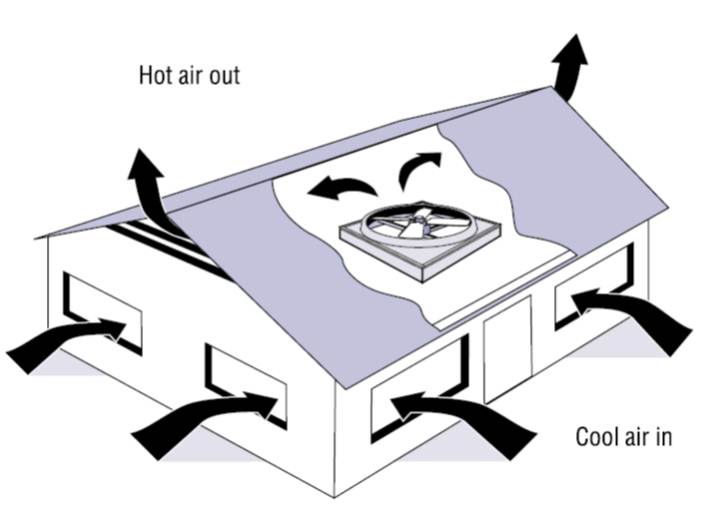
Whole-house fans depressurize the house and can cause issues with gas- or propane-fired equipment. If the home has any gas appliances with a standing pilot light or that are not sealed combustion with the combustion air ducted from the outside, a whole-house fan should not be used. Furnaces without a standing pilot light are acceptable If a switch is installed to prevent the whole-house fan from operating simultaneously with the furnace (DOE 1999). It is important that windows are open and fireplace dampers are closed before turning on the fan.
Whole-house fans must be carefully installed such that the ceiling remains well-sealed and insulated when the fan is not in use. Poorly installed whole-house fans can waste large amounts of energy in the winter. These details are not challenging for newer designs, but are a particular issue for traditional whole-house fan designs.
Noise can also be a concern. Choosing a fan with a curved or coiled flexible duct section between the fan and the occupied space can significantly reduce noise (Figure 32). A fan with multiple or variable operating speeds allows noise control as well as airflow control. Finally, as with wind-driven ventilation, whole-house fans can bring in pollen, dust, humid air, smoke, or pollution, and can be a security concern due to open windows.
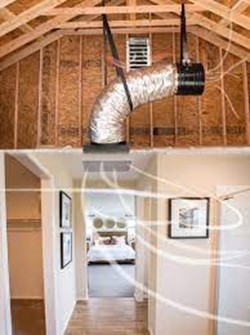
Keys to successful implementation of whole-house fans include the following recommendations:
- Size the fan properly.
- Install the fan such that it is well air-sealed and insulated when not in use.
- Select a fan with multiple speeds and/or noise control features.
- Locate the fan in a high, central location.
- Ensure windows are dispersed around the house.
- Provide free airflow between windows and the fan.
- Ensure windows and openings are easily accessible and convenient to operate.
- Provide sufficient window area for good airflow.
- Provide sufficient attic vent area for good exhaust airflow.
- Protect windows from rain using eaves, overhangs, or porches.
- Do not install unsealed combustion appliances in the home or attic.
See the Whole-House Fans guide for specific guidance on the above recommendations.
Ceiling Fans
Ceiling fans are a very effective option for providing sufficient air speeds for comfort ventilation when enough natural wind is not available. Unlike wind or whole-house fans, ceiling fans can be used with the windows closed to supplement air-conditioning (Figure 33). They do not bring in pollen, dust, smoke, pollution, or humid air, and they do not have the same air-leakage concerns that whole-house fans do when heating or air-conditioning. Ceiling fans offer significant flexibility and energy savings because only rooms that are occupied need to have a fan running.
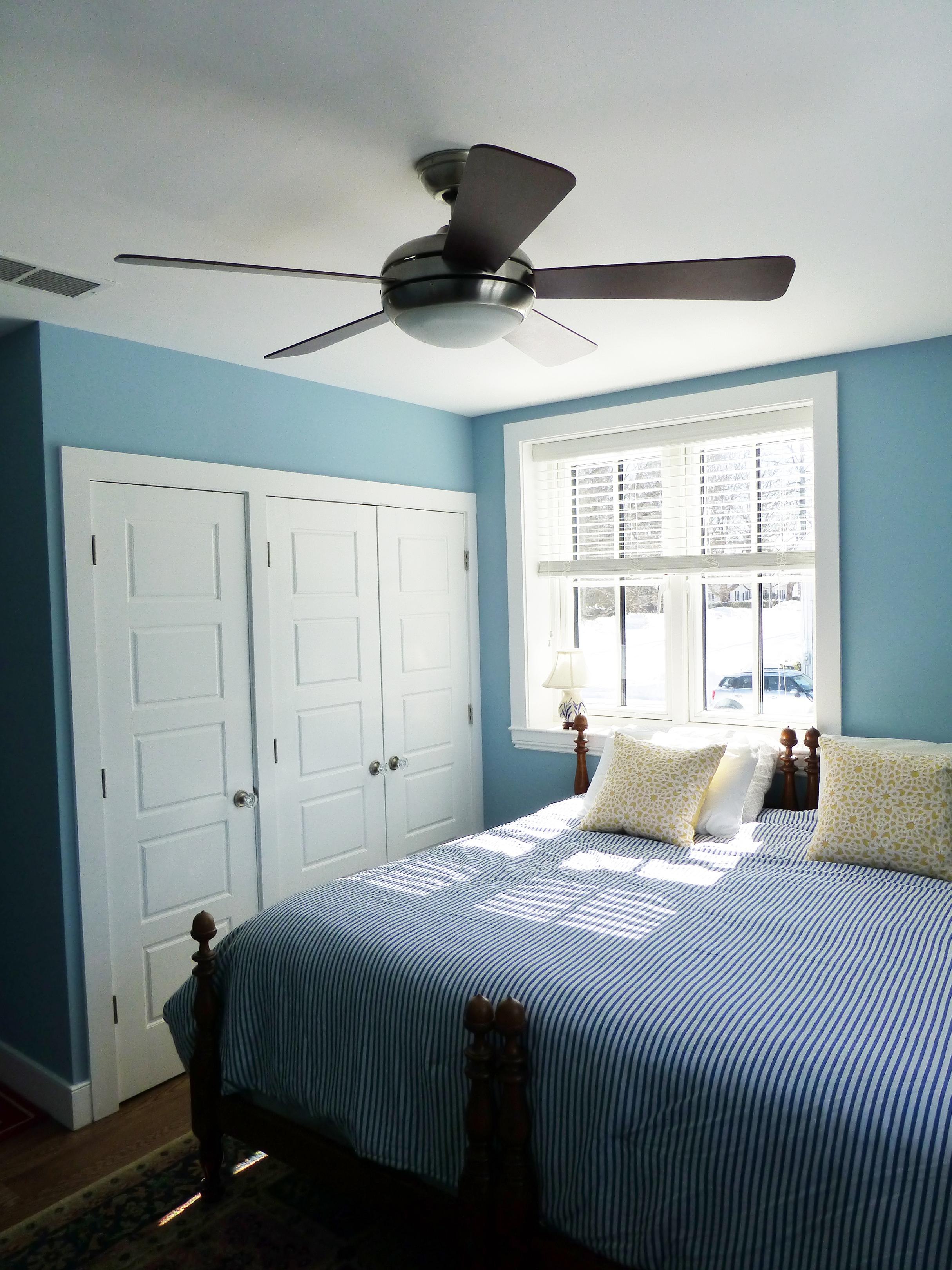
The target air speeds for comfort ventilation are very achievable for a room with a ceiling fan. At 50 FPM to 150 FPM, the cooling effect of moving air is about 2°F to 5°F (See Figure 12 above). Higher speeds may rustle papers or cause annoyance. Figure 34 shows that the air speeds generated by a typical ceiling fan are within the ideal range for comfort.
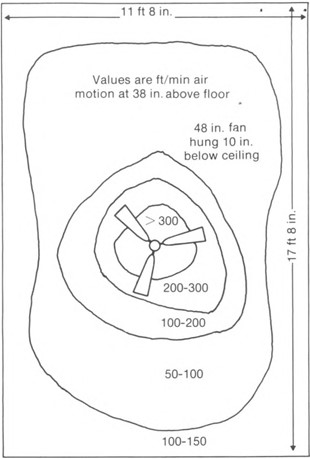
Ceiling fans cannot drive a night flush strategy because they do not bring in outside air. However, they can be used to enhance the strategy by providing additional cooling effect during the day. Running ceiling fans when doors and windows are closed during the day can extend the period of comfort inside the house. Ceiling fans can also help at night by increasing the rate that the interior mass of the house can cool down (Figure 35). The ideal strategy would be to operate ceiling fans in occupied areas once the house starts to warm up in the afternoon, then operate them throughout the house as needed during night flush.
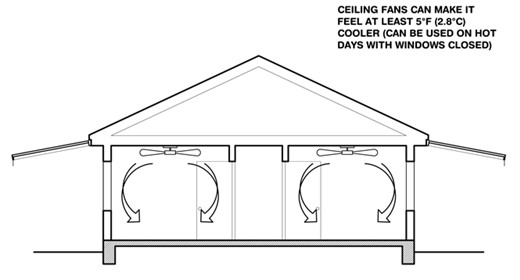
Keys to success with ceiling fans include the following recommendations:
- Size the fan per manufacturer guidance.
- Provide ceiling heights which can accommodate ceiling fans.
- Locate each fan centrally within a room.
- Select a high-efficiency unit (ENERGY STAR).
- Select a fan with multiple speeds.
- Consider controls such as occupancy sensors or timers.
See the Ceiling Fans (Energy Star) guide for more information.
Kitchen and Bathroom Exhaust Fans
Kitchen and bathroom exhaust fans should be incorporated into any cooling strategy. They are low energy consumers and provide very effective localized exhaust cooling at the hottest and most humid locations in the house. They do not produce enough air speed for comfort ventilation, however, and are weak contributors to a whole-house exhaust cooling or night flush strategy. See the Bathroom Exhaust Fans guide, Kitchen Exhaust Fans guide, and the Continuously Operating Ventilation and Exhaust Fans guide for more information.
Evaporative Cooling
Evaporation as a cooling source is very common and is most effective in dry climates. It can be used via commercial evaporative coolers or through completely passive means. Evaporative cooling has physical limits, however: the more hot and humid the environment is, the less effective evaporative cooling can be.
Evaporative Coolers
Evaporative coolers are a practical and effective cooling solution in very dry climates (Figures 36 and 37). They use a fraction of the energy of typical air conditioning units. Direct evaporative coolers (swamp coolers) add moisture to the airstream entering the home, while indirect evaporative coolers do not.
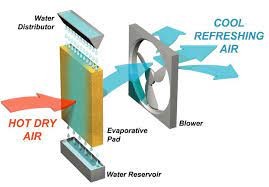
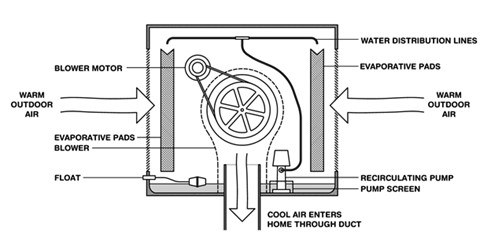
Direct and indirect evaporative coolers work best where the summertime design wet bulb temperature is below 70°F (LBNL 2007). In Figure 38, this region is shaded purple and indicated as “A”. In Region “B” (blue), direct evaporation will work only moderately well, but indirect evaporative cooling can be used as an effective pre-cool mechanism. In region “C”, where wet bulb temperatures are above 75°F, direct evaporative coolers are not appropriate, but indirect evaporative coolers could be used as a pre-cool mechanism.
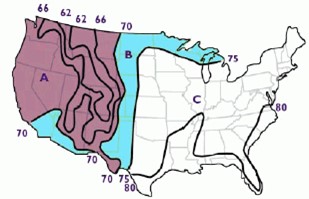
Direct evaporative coolers are typically sized to provide 20 to 40 air changes per hour (ACH) (DOE 2022). This means a 2,000-ft2 house with 8-foot ceilings would use a unit sized to provide about 5,000 to 10,000 CFM. The house will need to have at least 1 to 2 ft2 of net free window or vent area for each 1,000 CFM of evaporative cooler capacity. Evaporative coolers with a vent-only option can operate in a similar fashion to whole-house fans for exhaust cooling and night flush strategies. Power usage will likely be higher than a whole-house fan, however, due to airflow restrictions from ductwork and the filter.
Because direct evaporative coolers rely on large amounts of outdoor air, systems should not be operated when there is smoke or pollution in the air unless the air is well-filtered.
Consider the following recommendations when utilizing commercial evaporative coolers:
- Install in a dry climate where the summer design wet bulb temperature is below 70°F .
- Properly size the unit (20 to 40 air changes per hour).
- Maintain the unit regularly.
- Ensure there is a reliable water supply.
See the Evaporative Cooling Systems guide for more information.
Passive Cool Towers
“Cool towers” use evaporation to cool outside air, which creates a downdraft in the tower (Figure 39). This downdraft passively pushes cool, moist air into a building. Air movement can be enhanced using a solar chimney elsewhere in the building to draw air out during the daytime. These systems do not require any electricity or wind to operate. However, wind coming from the wrong direction could reduce or even reverse the airflow in the tower and prevent cooling. Similarly, solar heating of the tower could slow or reverse the air flow direction. As compared to a commercial evaporative cooler, these systems save fan energy but are less controllable and more costly to build. The upfront expense of building a cool tower instead of installing a commercial evaporative cooler would be difficult to justify in most situations based on fan energy costs alone.
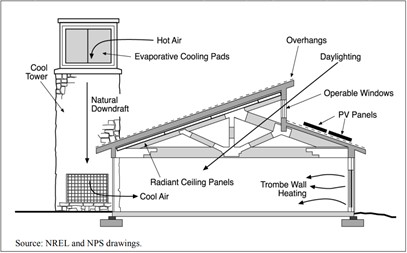
Other Evaporative Cooling Methods
Many other innovative evaporative methods have been used, such as roof spray systems, damp sheets hung in window openings, decorative water features inside the house, and outside water features or misters located in front of ventilation inlets (Figure 40). Any such system would almost certainly provide some cooling. Whether or not the cooling it provides is worth the upfront effort would have to be assessed on a case-by-case basis.
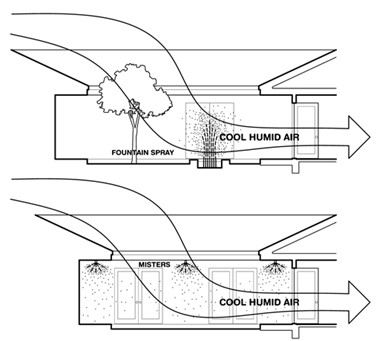
Ground Cooling
The ground, even just a few feet below grade, is usually significantly cooler than summertime air temperatures. The difference is most significant during heat waves, when the air warms quickly while the ground stays stable. These cooler temperatures, along with the enormous thermal mass of the ground, can be utilized to help keep a house cooler.
Ground temperatures near the surface fluctuate with the seasons, though there is a time lag such that peak ground temperatures can happen months after peak air temperatures. Deeper earth fluctuates less, and at depths of about 30 feet or more the temperature of undisturbed earth remains relatively stable at a temperature near to the local annual average temperature (or a few degrees higher). The colder the annual average temperature of a region is, the greater the potential for earth cooling. Figure 41 provides annual average temperatures throughout the contiguous United States.
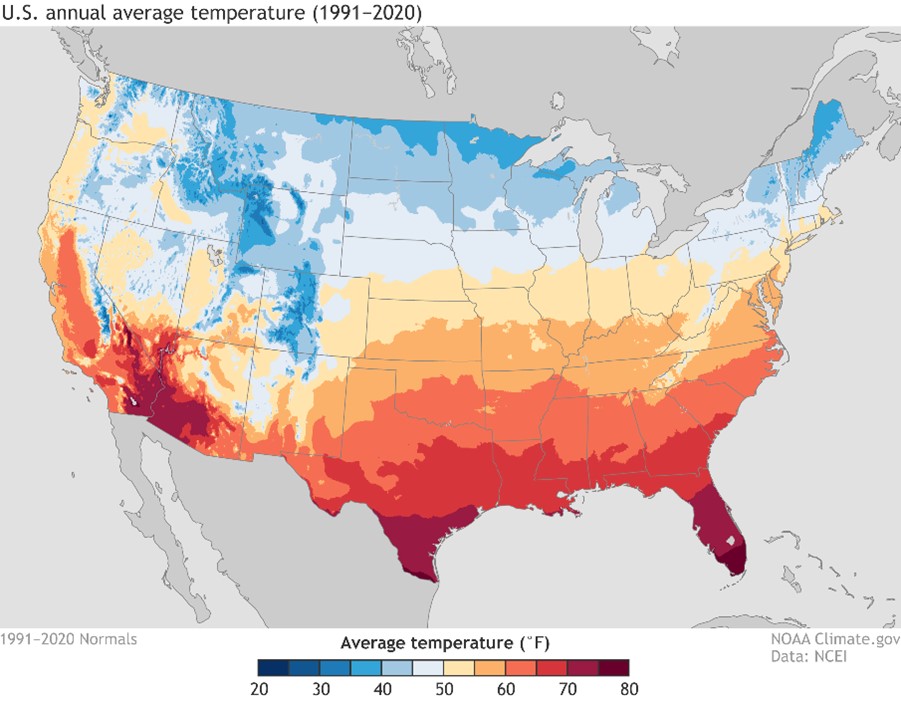
Basement Construction
Including a finished basement as part of a home can create an effective cool zone. The walls and floor of a basement are exposed to cooler ground temperatures rather than hot outdoor air temperatures. Basements also typically have few or no windows. With no solar heat gains and limited or no heat gain through the walls or slab, the only real sources of heat for a basement are internal loads, ventilation air, and air exchange with the floor above.
Basements have the additional advantage of being the lowest floor in the house, where cool air tends to settle. To keep the basement as a cool zone in a house, air exchange between the upper floors and the basement should be minimized. Night flush can be implemented, but introducing warm outdoor air for comfort ventilation is generally not recommended. Ceiling fans can be used for comfort ventilation without bringing in outdoor air. Ventilation for indoor air quality should be provided through an ERV.
While basements can make excellent cool zones, their ability to provide cooling is limited. The temperature of the soil surrounding a basement will eventually warm up to within a few degrees of the temperature inside the basement. These temperatures are low enough to virtually eliminate heat gain, but are not cool enough to provide substantial additional cooling to the rest of the home. Even if ground temperatures near the basement did stay cold, wall and slab insulation limit the basement’s access to ground cooling. It is not recommended to attempt to increase ground cooling by reducing insulation levels, however.
A dehumidifier in the basement may be necessary to keep humidity at reasonable levels. This is especially true for non-air-conditioned houses in humid climates. Because the basement is the naturally coolest place in the house, it will also have the highest humidity levels regardless of how well it has been waterproofed. A heat pump water heater installed in a basement may provide most or all of the cooling that is needed, as well as some dehumidification.
Keys to success when using a basement as a dedicated cool zone include the following recommendations:
- Properly waterproof and insulate the basement.
- Minimize air exchange between upper floors and the basement.
- Ensure effective external shading of any windows.
- Do not over-ventilate, and use energy recovery ventilation (ERV).
- Install a dehumidifier or dehumidification system.
- Install a heat pump water heater that rejects cooling into the basement.
- Use ceiling fans or other circulation fans to provide comfort ventilation without introducing outdoor air or air from upper floors.
Earth Tubes
Earth tubes are air ducts buried in the ground (Figure 42). The ground cools the air as it is drawn through the earth tubes by a fan. Earth tubes can be open-loop or closed-loop. Open-loop systems are used to cool outside air before it is brought into the house, usually to pre-cool ventilation air. Closed-loop systems simply recirculate and cool the inside air.
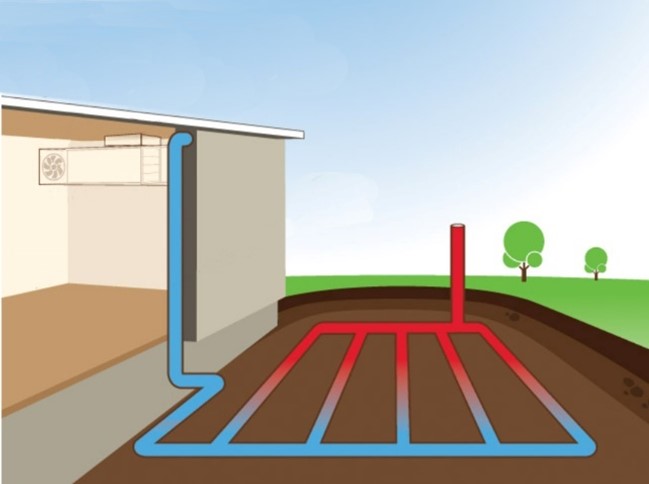
Earth tubes can provide legitimate cooling or ventilation pre-cooling, but their application is limited. Even an ideal system could only provide air as cold as the earth temperature at the depth it is buried. During the summer, this temperature can be expected to be higher than the annual averages shown in Figure 41, by about 10°F at typical installation depths. As systems are used over a period of days and weeks, the earth around the tubes warms up, raising the temperature of the air they can supply even more. Finally, limitations in the heat transfer effectiveness between the air and the tube further reduce the cooling ability of earth tubes. These effects combine such that an earth tube system could easily be expected to provide supply air in the summer that is 15°F or more above the local annual average soil temperature.
For these reasons, earth tube applications for cooling a house are limited to colder regions. The cooling provided by a system in warm or mild regions may not be worth the cost and effort of its installation. Pre-cooling of ventilation air, however, may be more viable as an energy-saving measure. When used for ventilation pre-conditioning, earth tubes can also act as a pre-heating mechanism for ventilation air in winter, as the ground temperatures at this time will be warmer than outdoor air temperatures. There will be times when the earth tubes could be detrimental to energy consumption (generally spring and fall). During early spring, for instance, the ground temperature around the tubes could easily be colder than the outdoor air, while the house is still calling for heat. During these times it would be beneficial to bypass the earth tube system and simply bring ventilation air directly in from the outside.
Tubes should be buried at least 5 to 10 feet deep in order to take advantage of earth temperatures, and the ground above them should be well-shaded (Abrams 1986). Greater depths will provide better performance but will also drive costs higher. Tube length and surface area must be sufficient for adequate heat transfer.
Humidity and air quality are a concern when using earth tubes. The relative humidity of the air rises inside the tubes, potentially creating an ideal environment for mold and mildew. Quality filtration at the outlet of the tube system can mitigate these concerns. Radon intrusion is an additional concern, so tubes must be well-sealed.
Earth tube technology has been used with varying success over the last several decades, but has not yet proven to be a consistently effective or economic alternative to other cooling methods. The best applications are for ventilation pre-conditioning or partial cooling for emergency backup. Most earth tube applications are still experimental (Figure 43).
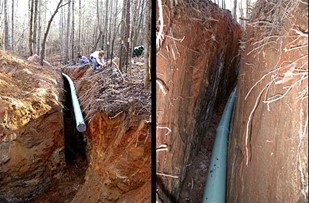
Recommendations for utilizing earth tubes include the following:
- Install in a cold climate with low ground temperatures.
- Bury tubes to a sufficient depth (at least 5-10 ft).
- Ensure ample tube length and surface area for adequate heat transfer.
- Space tubes sufficiently far apart to reduce ground-warming.
- Ensure effective shading of the ground surface above the tubes.
- Ensure tubes are well-sealed.
- Monitor supply air humidity and filter the air entering the home.
Radiative Cooling
Thermal radiation to the sky and outer space can be a source of cooling, particularly at night. Outer space is extremely cold. The atmosphere insulates the earth from the cold outer space, but not completely. Some of the thermal radiation emitted from objects such as a house passes easily through the atmosphere, which in turn has a cooling effect on the house. This cooling effect acts on all buildings at all times of day and night, but it is difficult to control and is often overshadowed by more powerful modes of heat transfer like solar radiation.
It is possible to intentionally manipulate this effect as a cooling source, but most methods are unwieldy or not realistic. Most systems are experimental or have not proven to be cost-effective (Figure 44). However, advancements continue to be made in this area, and viable systems may be developed in the future. Thermal radiation to outer space is more effective in dry climates because higher humidity and clouds can reduce or nearly eliminate the amount of thermal radiation that can pass through.
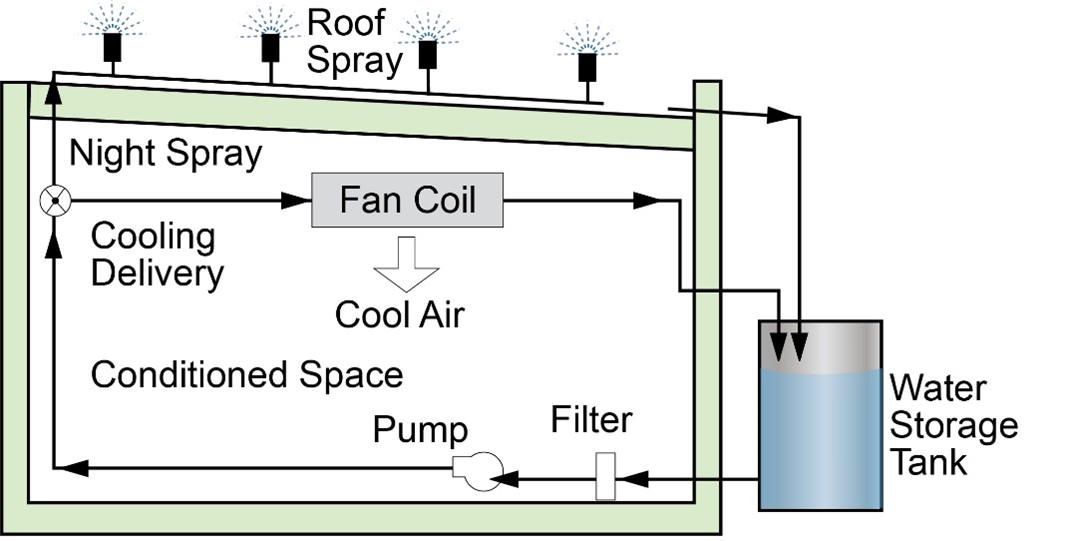
Heat Pump Water Heaters
Heat pump water heaters (HPWH) are not only highly efficient at providing hot water, but they also produce cooling and dehumidification to the surrounding air as a waste product. As a waste product requiring no additional energy input, this can be considered zero-energy cooling. This is essentially a form of air-conditioning that can be used intentionally to provide very limited and uncontrolled space cooling. More hot water consumption will result in more cooling.
The amount of cooling produced by a heat pump water heater will vary greatly depending on operation mode, hot water consumption, usage patterns, climate, and where the unit is located. As a reference, a heat pump water heater might produce around 6,000 – 10,000 BTUs of cooling per day for a typical three-bedroom house during the summer (Colon et al. 2016 ). This is less than 1/20th of the amount of cooling that would be provided by a typical window A/C unit running at full capacity all day and all night.
This amount of cooling may seem insignificant when considering an entire house, but in a small, interior, unventilated room or basement this amount of cooling could provide welcome relief. In a highly insulated and air-sealed home, the effect would be much more noticeable.
HPWHs are most useful in hot and humid environments. They provide one of the only zero-additional-energy opportunities for dehumidifying and actually lowering the temperature of the air. Using comfort ventilation throughout the house while having a small isolated “cool room” served by the HPWH would be a viable strategy in hot and humid regions. Because they require electricity to operate, they would not be a source of cooling during a power outage.
Several HPWH manufacturers make ducting kits for their units. These kits allow the HPWH to supply its waste cooling to a different room than where the water heater is located (Figure 45). If the unit is ducted to the outside, no cooling will be provided to the space.
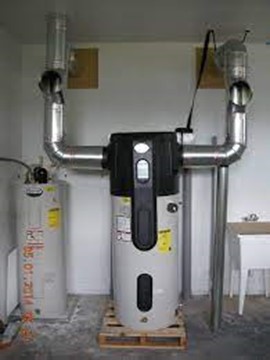
Success
The first key to successful implementation of passive and low-energy cooling is to focus on minimizing heat gain. Other keys include clearly defining the goals of the strategies, using climate-appropriate measures, and having realistic expectations. Keep strategies simple and convenient, using proven methods.
During an extreme heat event without air-conditioning or during power loss, the focus of passive cooling moves from comfort to safety. Most of the passive measures described in this guide can help to some degree in nearly any climate. When the focus is simply on safety rather than comfort, the temperature and humidity restrictions applied to the various passive cooling techniques can be stretched significantly.
Climate
Basic recommendations for passive and low-energy cooling approaches are provided below for different climate regions. The climate zones referenced are based on the map in Figure 1.
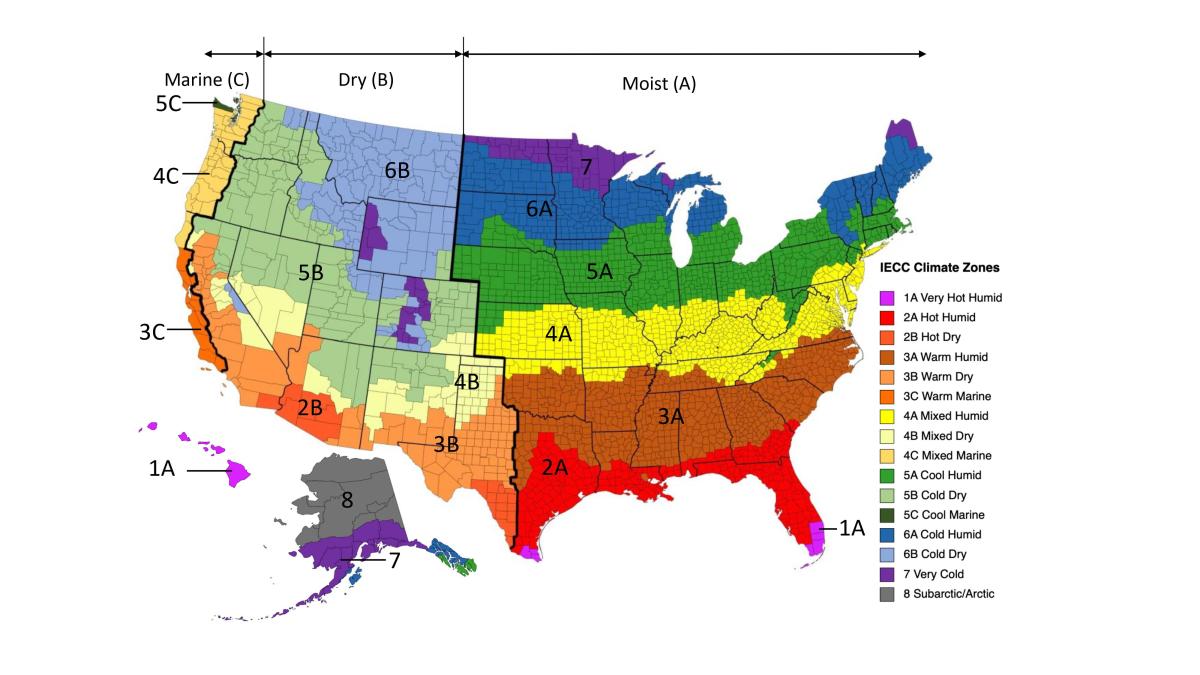
Passive and Low Energy Cooling Recommendations per Climate Region
Hot-Humid/Warm-Humid (IECC Climate Zones 1A, 2A, 3A)
In the hottest and most humid climates, cooling strategies should generally focus on effective shading and comfort ventilation day and night. Exhaust cooling can also be utilized. Heat pump water heaters should be used to provide some waste cooling and dehumidification. The cooling and dehumidification effects of heat pump water heaters are more valuable in this climate than any other. Low thermal mass construction will allow lower evening and nighttime temperatures, when occupancy is often highest, and when sleep can be disturbed by high temperatures.
If mechanical air-conditioning is to be used, then windows should be closed and means of bringing in outdoor air should be limited to ventilation for indoor air quality. Comfort ventilation can be achieved by ceiling fans to supplement the air-conditioning and allow a more conservative thermostat set point. If using a whole-house fan, the best approach in humid climates is to keep the air-conditioning off and use the whole-house fan when the seasonal temperatures allow (generally spring and fall). Once the summer becomes hot enough to call for extended air-conditioning, the whole-house fan should be kept off until seasonal temperatures cool off again. Shading, heat pump water heaters, and low thermal mass are all appropriate for use in conjunction with mechanical air-conditioning.
A simple approach in this climate would be as follows:
- Use low-mass construction.
- Insulate, air seal, and select windows per local code or a higher standard such as DOE ZERH or ENERGY STAR .
- Shade east/west windows with landscaping (most preferred), exterior shutters (preferred), or interior blinds (least preferred). It is recommended to shade north/south windows as well.
- Locate operable windows all around the house, providing free flow between.
- Install ceiling fans for comfort ventilation.
- Consider installing a whole-house fan for exhaust cooling when wind is calm.
- Install exhaust fans near showers, cooking, and at high points in the home.
- Install a heat pump water heater.
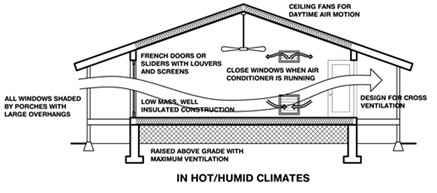
Hot-Dry/Warm-Dry (IECC Climate Zones 1B/2B/3B)
In hot-dry climates, cooling strategies should generally focus on effective shading, evaporative cooling, and night flush. Evaporative cooling is very effective in this region. During the hottest parts of the summer, evaporative cooling may not be capable of bringing the temperature down to comfort conditions, but the cooling relative to outside temperatures will be very significant. Night flush can be effective during the milder parts of spring, summer, and fall. Comfort ventilation via ceiling fans will benefit both evaporative cooling and night flush strategies. Heat pump water heaters are appropriate to provide some waste cooling.
If mechanical air-conditioning is to be used, it could be effectively coordinated with a night flush strategy for part of the season. Ceiling fans and heat pump water heaters would effectively supplement A/C, but evaporative cooling should not be used when using mechanical air-conditioning.
A simple approach in this climate would be:
- Insulate, air seal, and select windows per local code or a higher standard such as DOE ZERH or ENERGY STAR.
- Shade east/west windows with landscaping (most preferred), exterior shutters (preferred), or interior blinds (least preferred). It is recommended to shade north/south windows as well.
- Locate operable windows all around the house, providing free flow between.
- Install an evaporative cooler.
- Install ceiling fans.
- Consider installing a whole-house fan or using the evaporative cooler as a whole-house fan.
- Use exhaust fans near showers, cooking, and at high points in the home.
- Use a heat pump water heater.
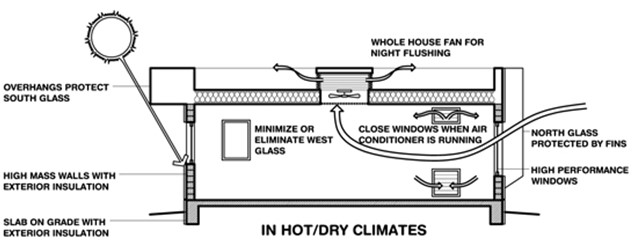
Mixed-Humid/ Cool Humid (IECC Climate Zones 4A, 5A)
In mixed and cool humid climates, passive and low energy strategies should generally focus on effective shading, comfort ventilation, and partial night flush. Night flush concepts cannot be relied upon to maintain cool temperatures during the warmest parts of the summer in this region. Low thermal mass construction may be best to allow more rapid nighttime cool-down in regions where nighttime temperatures do not consistently dip below about 67°F throughout the summer. High mass construction and a night flush strategy may be appropriate in areas where the nighttime temperatures are not higher than about 67°F, and the difference between the daytime high temperature and the nighttime low is greater than about 25°F. Heat pump water heaters are appropriate to provide some waste cooling and dehumidification, though this will add load to the heating system in winter. Basement construction can be effective in this region. It may not be advisable to site a house to increase wind exposure as this will increase heating energy consumption in the winter.
If mechanical air-conditioning is to be used, then windows should be closed and means of bringing in outdoor air should be limited to ventilation for indoor air quality. Comfort ventilation can be achieved by ceiling fans to supplement the air-conditioning and allow a more conservative thermostat set point. If using a whole-house fan, the best approach in humid climates is to keep the air-conditioning off and use the whole-house fan when the seasonal temperatures allow (generally spring and fall). Once the summer becomes hot enough to call for extended air-conditioning, the whole-house fan should be kept off until seasonal temperatures cool off again. Shading and heat pump water heaters are appropriate for use in conjunction with mechanical air-conditioning. A night flush strategy could be carefully coordinated with the use of an air conditioner during the cooler and drier parts of the season.
A simple approach in this climate would be:
- Insulate, air seal, and select windows per local code or a higher standard such as DOE ZERH or ENERGY STAR.
- Shade east/west windows with landscaping (most preferred), exterior shutters (preferred), or interior blinds (least preferred). Use overhangs or deciduous trees to shade south windows in summer but let sunlight in in winter.
- Locate operable windows all around the house, providing free flow between.
- Install ceiling fans for comfort ventilation.
- Consider installing a whole-house fan for exhaust cooling or night flush.
- Install exhaust fans near showers, cooking, and possibly at high points in the home.
Mixed-Dry/Cool Dry (IECC Climate Zones 4B, 5B)
In mixed-dry and cool-dry climates, passive and low energy strategies should generally focus on effective shading, night flush, and evaporative cooling. Night flush can be highly effective in this region, and high mass construction may well be justified. Evaporative cooling can be used as the primary cooling source or as a backup to night flush. Heat pump water heaters are appropriate to provide some waste cooling, though this will add load to the heating system in winter. Basement construction can be effective in this region. It may not be advisable to site a house to increase wind exposure as this will increase heating energy consumption in the winter.
If mechanical air-conditioning is to be used, it could be effectively coordinated with a night flush strategy for most of the season. Ceiling fans and heat pump water heaters would effectively supplement A/C, but evaporative cooling should not be used when air-conditioning.
A simple approach in this climate would be:
- Insulate, air seal, and select windows per local code or a higher standard such as DOE ZERH or ENERGY STAR.
- Shade east/west windows with landscaping (most preferred), exterior shutters (preferred), or interior blinds. Use overhangs or deciduous trees to shade south windows in summer but let sunlight in in winter.
- Locate operable windows all around the house, providing free flow between.
- Install ceiling fans.
- Install an evaporative cooler and/or a whole-house fan and thermal mass.
- Install exhaust fans near showers, cooking, and possibly at high points in the home.
Warm Marine/Mixed Marine (IECC Climate Zones 3C, 4C)
In marine climates, passive and low energy strategies should generally focus on effective shading, comfort ventilation, night flush, and evaporative cooling. Full or partial night flush can be very effective in this region, and high mass construction can be justified where the nighttime temperatures are not higher than about 67°F, and the difference between the daytime high temperature and the nighttime low is greater than about 25°F. Evaporative cooling can be used as the primary cooling source in many areas or as a backup to night flush. Heat pump water heaters are appropriate to provide some waste cooling, though this will add load to the heating system in winter.
If mechanical air-conditioning is to be used, it could be effectively coordinated with a night flush strategy for most of the season. Ceiling fans and heat pump water heaters would effectively supplement A/C, but evaporative cooling should not be used when air-conditioning.
A simple approach in this climate would be:
- Insulate, air seal, and select windows per local code or a higher standard such as DOE ZERH or ENERGY STAR.
- Shade east/west windows with landscaping (most preferred), exterior shutters (preferred), or interior blinds. Use overhangs or deciduous trees to shade south windows in summer but let sunlight in in winter.
- Locate operable windows all around the house, providing free flow between.
- Install ceiling fans.
- Install a whole-house fan and consider incorporating thermal mass.
- Consider an evaporative cooler.
- Install exhaust fans near showers and cooking.
Cold-Humid/Very Cold (IECC Climate Zones 6A, 7, 8)
In cold-humid and very cold climates, cooling strategies should generally focus on effective shading, comfort ventilation, and night flush. Night flush can be highly effective in this region, but the short cooling season makes high mass construction hard to justify unless it is part of the heating strategy as well as the cooling strategy. Basement construction and ground-cooling can be effective in this region. It is not advisable to site a house to increase wind exposure as this will increase heating energy consumption in the winter.
If mechanical air-conditioning is to be used, then windows should be closed and outdoor air should be limited to ventilation for indoor air quality. Comfort ventilation can be achieved by ceiling fans to supplement the air-conditioning and allow a more conservative thermostat set point. A night flush strategy could be carefully coordinated with the use of an air conditioner during the cooler and drier parts of the season. If using a whole-house fan, the best approach in humid climates is to keep the air-conditioning off and use the whole-house fan when the seasonal temperatures allow (generally spring and fall). If the summer becomes hot enough to call for extended air-conditioning, the whole-house fan should be kept off until seasonal temperatures cool off again.
A simple approach in this climate would be:
- Insulate, air seal, and select windows per local code or a higher standard such as DOE ZERH or ENERGY STAR.
- Shade east/west windows with landscaping (most preferred), exterior shutters (preferred), or interior blinds. Keep south windows unshaded in winter.
- Locate operable windows all around the house, providing free flow between.
- Install ceiling fans.
- Properly install a whole-house fan and consider incorporating thermal mass.
- Install exhaust fans near showers and cooking.
- Incorporate a full, finished basement.
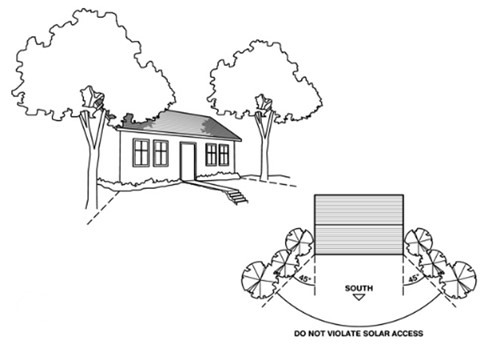
Cold-Dry (IECC Climate Zone 6B)
In the cold-dry climate, passive and low energy strategies should generally focus on effective shading, comfort ventilation, night flush, and evaporative cooling. Night flush can be highly effective in this region, but the short cooling season makes high mass construction hard to justify unless it is part of the heating strategy as well as the cooling strategy. Evaporative cooling is very effective in this area. Basement construction and ground-cooling can also be effective in this region. It is not advisable to site a house to increase wind exposure as this will increase heating energy consumption in the winter.
If mechanical air-conditioning is to be used, it could be effectively coordinated with a night flush strategy for most of the season. Ceiling fans would effectively supplement A/C, but evaporative cooling should not be used when air-conditioning.
A simple approach in this climate would be:
- Insulate, air seal, and select windows per local code or a higher standard such as DOE ZERH or ENERGY STAR.
- Shade east/west windows with landscaping (most preferred), exterior shutters (preferred), or interior blinds. Keep south windows unshaded in winter.
- Locate operable windows all around the house, providing free flow between.
- Install ceiling fans.
- Consider installing an evaporative cooler or a whole-house fan and thermal mass.
- Use exhaust fans near showers and cooking, and possibly at high points in the home.
- Incorporate a full, finished basement.
Training
Compliance
Compliance
The Compliance tab contains both program and code information. Code language is excerpted and summarized below. For exact code language, refer to the applicable code, which may require purchase from the publisher. While we continually update our database, links may have changed since posting. Please contact our webmaster if you find broken links.
2022 California Energy Commission Title 24 Energy Code Single-Family Residential Compliance Manual
Section 4.7.10 Ventilation Cooling. Discusses ventilation cooling (exhaust cooling and night flush). Provides helpful background on strategies and potential savings.
Section 4.7.10.1: Discusses whole-house fans specifically.
Section 4.7.10.3 (Section 150.1 (c) 12 in 2016). Discusses prescriptive requirements and equipment standards for whole-house fans. States that whole-house fans are required in California Climate Zones 8-14 (these are different from the IECC climate zones). Whole-house fans must be listed in the HVI Certified Products Directory. Whole-house fans must have a listed airflow of at least 1.5 CFM/ft2. Attic vents must be at least 1 ft2 of free area per 750 CFM of airflow.
2020 Florida Building Code, Energy Conservation, 7th Edition
Section 405.7 states that, when using the simulated performance alternative, credit may be claimed in the software compliance calculation for radiant barriers, cool roofs, cross ventilation, whole-house fans, and ceiling fans.
Section 405.7.1 provides criteria for homes claiming credit for attic radiant barriers.
Section 405.7.2 provides criteria for homes claiming credit for cool roofs.
Section 405.7.4 provides criteria for homes claiming credit for cross-ventilation. Specifically, operable openings must equal at least 12% of the floor area in primary living areas and main bedrooms, insect screens must be provided, at least two openings must be provided (cannot be located on the same wall unless properly designed wing walls are used).
Section 405.7.5 provides criteria for homes claiming credit for whole-house fans. Specifically, fans must be sized to provide a minimum of 20 ACH, must be sized to provide at least 3 CFM/ft2, and must have attic vents equal to at least 4 times the area of the ceiling cutout for the whole-house fan.
Section 405.7.6 provides criteria for homes claiming credit for ceiling fans. Specifically, fans should be installed in each bedroom and primary living areas. Guidelines for fan blade diameters are also provided based on the length of the longest wall in the room.
2009, 2012, 2015, 2018, and 2021 International Residential Code (IRC)
Chapter 15 Exhaust Systems. Describes requirements for exhaust ventilation systems, including whole-house exhaust systems.
Retrofit
It is best to incorporate passive and low-energy cooling strategies into a home when first built. However, there are many opportunities to retrofit an existing home. Some retrofits can make sense as stand-alone projects, while others would be best done as part of a larger retrofit or replacement project. The list below provides some possible stand-alone retrofits for minimizing heat gain or providing passive and low-energy cooling. They are shown in approximate order of least involved to most involved.
- Plant shade trees or bushes.
- Install ceiling fans (if ceiling height allows).
- Install a heat pump water heater.
- Add landscaping water features.
- Install bathroom and kitchen exhaust fans.
- Install exhaust fans at high points in the home.
- Add low-SHGC exterior storm windows.
- Install interior louvers or jump ducts for cross ventilation.
- Add exterior window shading such as shutters, blinds, solar shades, or awnings.
- Add blown-in attic insulation and/or radiant barrier.
- Install a whole-house fan.
- Add strategically placed windows or vents.
- Install an evaporative cooler and ductwork.
- Replace hard scaping and parking areas with light-colored, permeable surfaces.
- Replace existing roof with a cool roof (especially if attic or roof deck is unvented).
- Create a more open floor plan for cross ventilation.
More
More Info.
Access to some references may require purchase from the publisher. While we continually update our database, links may have changed since posting. Please contact our webmaster if you find broken links.